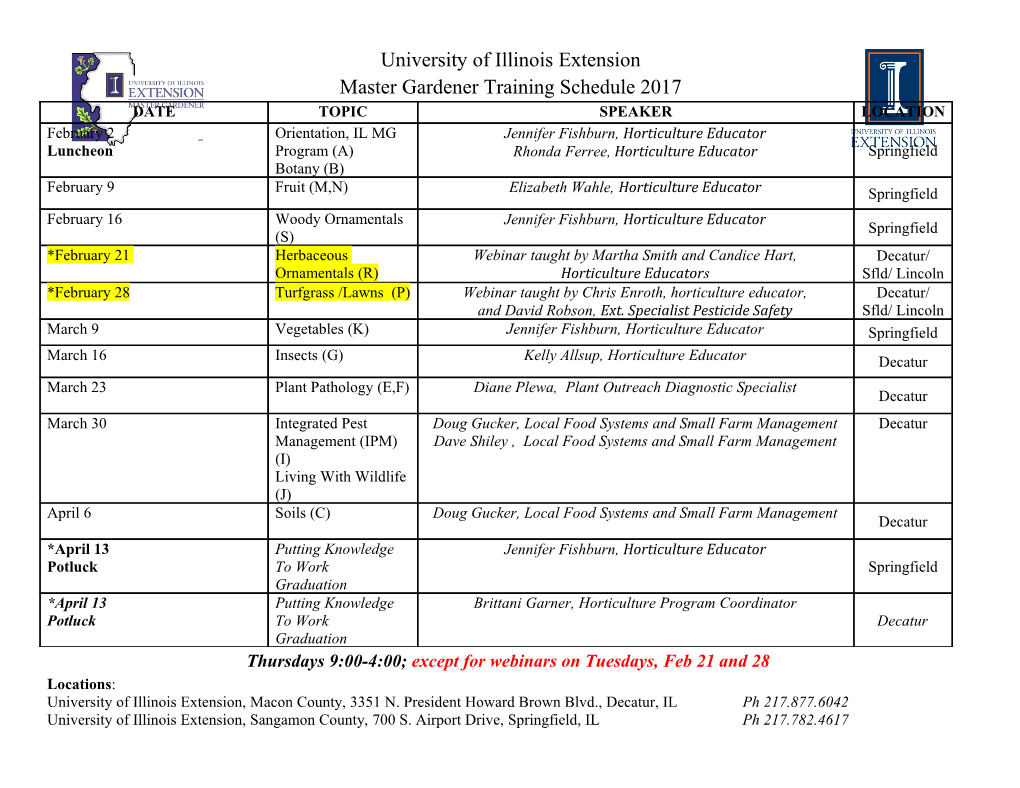
http://www.diva-portal.org This is the published version of a paper published in Journal of Breath Research. Citation for the original published paper (version of record): Ghorbani, R., Schmidt, F M. (2019) Fitting of single-exhalation profiles using a pulmonary gas exchange model: application to carbon monoxide Journal of Breath Research, 13(2): 026001 https://doi.org/10.1088/1752-7163/aafc91 Access to the published version may require subscription. N.B. When citing this work, cite the original published paper. Permanent link to this version: http://urn.kb.se/resolve?urn=urn:nbn:se:umu:diva-152093 J. Breath Res. 13 (2019) 026001 https://doi.org/10.1088/1752-7163/aafc91 PAPER Fitting of single-exhalation profiles using a pulmonary gas exchange OPEN ACCESS model—application to carbon monoxide RECEIVED 19 December 2018 Ramin Ghorbani and Florian M Schmidt ACCEPTED FOR PUBLICATION Department of Applied Physics and Electronics, Umeå University, SE-90187 Umeå, Sweden 8 January 2019 fl PUBLISHED E-mail: [email protected] 1 March 2019 Keywords: real-time breath gas analysis, carbon monoxide (CO), pulmonary gas exchange model, single-exhalation profile, laser absorption spectroscopy Original content from this work may be used under the terms of the Creative Commons Attribution 3.0 licence. Abstract Any further distribution of Real-time breath gas analysis coupled to gas exchange modeling is emerging as promising strategy to this work must maintain enhance the information gained from breath tests. It is shown for exhaled breath carbon monoxide attribution to the author(s) and the title of (eCO), a potential biomarker for oxidative stress and respiratory diseases, that a weighted, nonlinear the work, journal citation and DOI. least-squares fit of simulated to measured expirograms can be used to extract physiological parameters, such as airway and alveolar concentrations and diffusing capacities. Experimental CO exhalation profiles are acquired with high time-resolution and precision using mid-infrared tunable diode laser absorption spectroscopy and online breath sampling. A trumpet model with axial diffusion is employed to generate eCO profiles based on measured exhalation flow rates and volumes. The − concept is demonstrated on two healthy non-smokers exhaling at a flow rate of 250 ml s 1 during − normal breathing and at 120 ml s 1 after 10 s of breath-holding. The obtained gas exchange parameters of the two subjects are in a similar range, but clearly distinguishable. Over a series of twenty consecutive expirograms, the intra-individual variation in the alveolar parameters is less than 6%. After a 2 h exposure to 10±2 ppm CO, end-tidal and alveolar CO concentrations are significantly increased (by factors of 2.7 and 4.9 for the two subjects) and the airway CO concentration is slightly higher, while the alveolar diffusing capacity is unchanged compared to before exposure. Using model simulations, it is found that a three-fold increase in maximum airway CO flux and a reduction in alveolar diffusing capacity by 60% lead to clearly distinguishable changes in the exhalation profile shape. This suggests that extended breath CO analysis has clinical relevance in assessing airway inflammation and chronic obstructive pulmonary disease. Moreover, the novel methodology contributes to the standardization of real-time breath gas analysis. 1. Introduction sample contamination due to pre-concentration and storage procedures. An additional benefit of breath- During the past decade, the advent of novel analytical cycle-resolved detection is that single-exhalation pro- techniques has intensified the interest in real-time files contain spatiotemporal information about the gas detection of trace species in exhaled breath as alter- exchange in the respiratory tract. Coupled to suitable native to offline analysis [1, 2]. In the context of this mathematical models of gas exchange [3], this enables work, real-time breath gas analysis refers to controlled biomarker source discrimination and non-invasive online breath sampling and subsequent quantitative determination of physiological parameters, which can biomarker detection with sufficient measurement lead to improved data interpretation, a better under- time-resolution (usually sub-second) and precision to standing of the origin and biochemical pathways of accurately resolve individual breath cycles. Compared biomarkers and, eventually, to novel breath tests. to offline mixed- or end-tidal breath sampling, the The shape of an exhalation profile primarily advantages of theonline approach include fastresponse, depends on the locations of biomarker production the possibility for continuous (inline) breath monitor- and exchange in the respiratory tract (alveoli, airways, ing over longer time periods, and reduced risk for oral/nasal cavities), and the breath sampling © 2019 IOP Publishing Ltd J. Breath Res. 13 (2019) 026001 R Ghorbani and F M Schmidt conditions (e.g. exhalation flow rate and volume, In general, exhaled CO also depends on recent envir- inhaled concentration, body position) [3]. In general, onmental exposure, such air pollution and smoking compounds with low water/blood solubility will [28], and on the molecular diffusion properties in the exchange in the alveolar region, whereas highly water- respiratory tract, which may deviate from normal in soluble molecules will exchange in the airways. For diseased cohorts. For example, in patients with severe example, both carbon monoxide (CO) and nitric COPD, the CO diffusing capacity is significantly oxide (NO) have a low water solubility, but exhaled reduced [29]. Conventional end-tidal eCO analysis CO originates mainly from the alveoli, leading to high with electrochemical sensors cannot resolve a poten- end-tidal values [4], while exhaled NO to a large extent tial small airway contribution or assess pulmonary dif- stems from ambient air and nasal/airway production, fusion, which hampers the interpretation of eCO resulting in a characteristic maximum in the begin- concentrations outside the healthy population range. ning of the exhalation [3, 5]. High initial concentra- To add value to eCO diagnostics, a compact LAS ( ) tions can also be expected for ammonia NH3 , which sensor for sensitive real-time detection of CO in mostly originates from the oral cavity, but the excep- exhaled breath and ambient air has recently been tionally high water solubility and the propensity to developed [4, 30]. Moreover, a trumpet model with adsorption hamper quantitative detection and have so axial diffusion (TMAD) has been adapted to, for the far prevented the reliable measurement of NH3 exha- first time, simulate pulmonary gas exchange dynamics lation profiles [6]. and single-exhalation profiles of CO during systemic Real-time breath analysis is routinely performed elimination [31]. The exhalation profiles are calcu- ( ) in clinical practice only for carbon dioxide CO2 lated based on four parameters, namely the CO diffus- using miniature capnographs based on non-dispersive ing capacities and maximum fluxes in the airways and infrared (NDIR) spectroscopy. Compact optical set- the alveolar region. In that study, simulated and mea- ups can also be used for rapid measurement of the sured exhalation profiles have been visually compared ( ) other major breath species, oxygen O2 and water to roughly estimate the model parameters and predict ( ) [ ] vapor H2O 7 . However, more sophisticated analy- the equilibrium CO concentrations in the two tical techniques are needed for real-time analysis of the compartments. less abundant molecules. These methods include soft- In this work, a weighted, nonlinear least-squares ionization mass spectrometry (MS), such as selected- fit of the model solution at the mouth grid point to the fl [ ] ion ow tube and proton transfer reaction MS 1 , experimental CO exhalation profiles is used to extract [ ] electrospray ionization MS 8 , non-equilibrium dilu- the TMAD parameters. The purpose of applying a fit [ ] tion ion mobility spectrometry 5 and laser absorp- instead of a manual comparison, is to enable fast, reli- ( ) [ ] tion spectroscopy LAS 2, 9 . able and precise extraction of the model parameters, Mathematical models of pulmonary gas exchange including end-tidal CO, in a consistent way. Such in the respiratory tract were developed early on to strategy is little described in the context of breath gas enable determination of the fractional airway NO analysis, but can greatly contribute to the standardiza- [ ] contribution 10, 11 and to improve the interpreta- tion of (real-time) breath sampling and data evalua- [ ] [ ] fi tion of CO2 12 and ethanol 3 exhalation pro les. tion. Precise and systematic profile analysis may also Physiological modeling was also used to better under- lead to improved gas exchange models and a better [ ] stand short-term changes in exhaled isoprene 13 and understanding of the biomarker physiology. Normal [ ] acetone 14 concentrations. In most of these model breathing and BH expirograms from two healthy non- implementations, end-tidal biomarker levels were smokers are analyzed to demonstrate the novel computed and compared to experiments. Only a few approach. The inter- and intra-individual variations in fi attempts have been made to compare or t simula- the model parameters, and the influence of acute tions of entire expirograms to experimental real-time exposure to elevated CO levels on the parameters are [ – ] data 15 19 . In a recent work by Mountain et al least- scrutinized. Furthermore, using simulations, it is fi ( ) fi squares tting of
Details
-
File Typepdf
-
Upload Time-
-
Content LanguagesEnglish
-
Upload UserAnonymous/Not logged-in
-
File Pages14 Page
-
File Size-