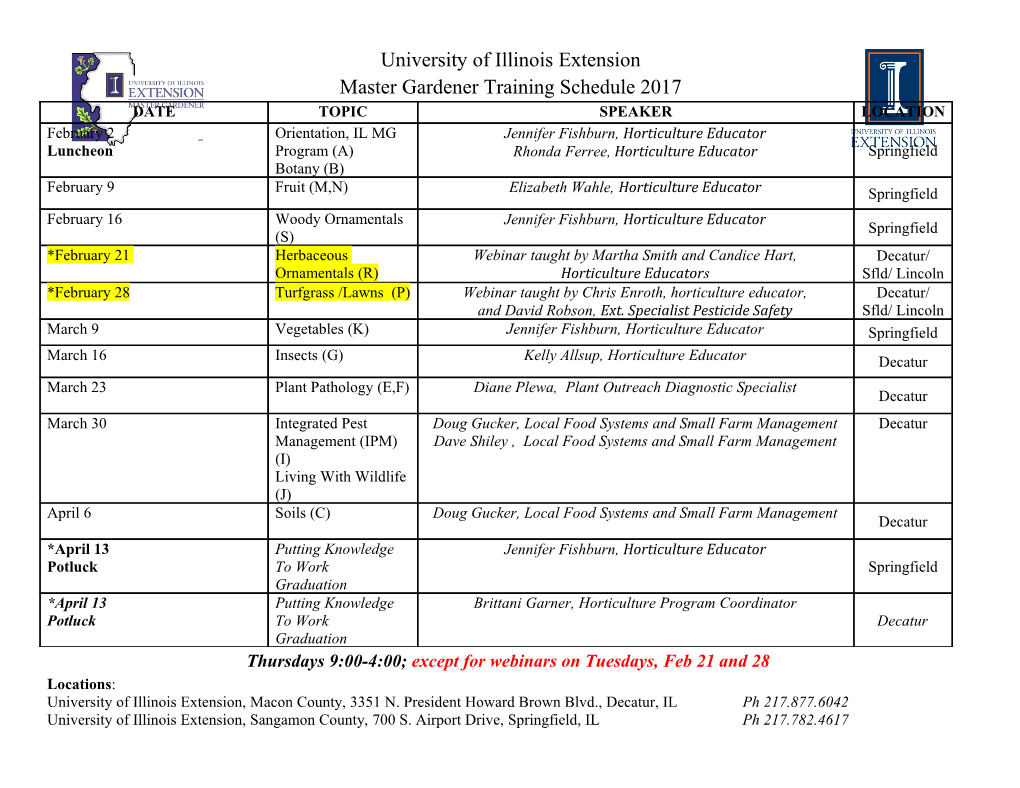
Proc. Natl. Acad. Sci. USA Vol. 96, pp. 8884–8889, August 1999 Biochemistry Crystal structure and mechanism of CO dehydrogenase, a molybdo iron-sulfur flavoprotein containing S-selanylcysteine HOLGER DOBBEK*†,LOTHAR GREMER‡,ORTWIN MEYER‡, AND ROBERT HUBER* *Max-Planck-Institut fu¨r Biochemie, Abteilung Strukturforschung, D-82152 Martinsried, Germany; and ‡Lehrstuhl fu¨r Mikrobiologie, Universita¨t Bayreuth, D-95440 Bayreuth, Germany Contributed by Robert Huber, May 27, 1999 ABSTRACT CO dehydrogenase from the aerobic bacte- requires conformational changes of M introduced through the rium Oligotropha carboxidovorans catalyzes the oxidation of binding of M to LS (unpublished data). The S subunit harbors ؉ CO with H2O, yielding CO2, two electrons, and two H . Its two [2Fe–2S] centers, which are proximal and distal, respec- crystal structure in the air-oxidized form has been determined tively, to the molybdenum center. They are traditionally des- to 2.2 Å. The active site of the enzyme, which contains ignated type I and II according to spectroscopic properties, but molybdenum with three oxygen ligands, molybdopterin- the assignment is controversial (8, 9). Recent experiments with cytosine dinucleotide and S-selanylcysteine, delivers the elec- M subunit-depleted CO dehydrogenase preparations assign trons to an intramolecular electron transport chain composed the proximal center to type I and the distal to type II of two types of [2Fe–2S] clusters and flavin-adenine dinucle- (unpublished data). otide. CO dehydrogenase is composed of an 88.7-kDa molyb- CO dehydrogenase has been grouped into the sequence doprotein (L), a 30.2-kDa flavoprotein (M), and a 17.8-kDa family of molybdenum hydroxylases (10). Other prominent iron-sulfur protein (S). It is organized as a dimer of LMS representatives of that family catalyze the oxidative hydroxy- heterotrimers and resembles xanthine dehydrogenase/oxidase lation of a diverse range of aldehydes and aromatic heterocy- in many, but not all, aspects. A mechanism based on a cles (9). The high-resolution structure of aldehyde oxidoreduc- structure with the bound suicide-substrate cyanide is sug- tase (Mop) from Desulfovibrio gigas has been solved and has gested and displays the necessity of S-selanylcysteine for the provided an insight into an active site of a molybdenum catalyzed reaction. hydroxylase (11). Mop and CO dehydrogenase both contain the MCD–molybdenum cofactor and the same [2Fe–2S] clus- CO is a trace gas and important scavenger for hydroxyl radicals ters. However, Mop is devoid of flavin and contains a single in the atmosphere. About one fifth of CO [115–230 Tg⅐yrϪ1 polypeptide chain, whereas CO dehydrogenase is a heterotri- (1)] is used by soil microorganisms (2, 3). CO has a short meric flavoprotein. lifetime within the soil, and consumption of atmospheric CO The CO dehydrogenase structural genes (cox)ofO. carbox- occurs mainly in the top few centimeters of the humus layer (O idovorans are clustered in the transcriptional order 5Ј-coxM- horizon), most likely under aerobic conditions. coxS-coxL-3Ј along with the Calvin cycle (cbb) and hydroge- Under aerobic conditions, CO can be used as the sole source nase (hox) genes on a 30-kb DNA segment of the 128-kilobase of carbon and energy by the carboxidotrophic bacteria, which megaplasmid pHCG3 (10, 12). CO dehydrogenase shows comprise a taxonomically diverse group of obligate or facul- significant sequence similarities to the two domains of Mop tative chemolithoautotrophic species (3, 4). Under anaerobic (10, 11). CoxL is 57% similar (25% identical) to amino acids conditions, CO is used with low affinity by a number of 175–907 of Mop, and CoxS is 74% similar (41% identical) to methanogenic archaea and acetogenic, sulfate-reducing, or amino acids 1–166 of Mop. phototrophic bacteria (3, 5). Generally, anaerobic CO dehy- drogenases are nickel-containing [4Fe-4S] proteins. CO dehy- MATERIALS AND METHODS drogenases of methanogens or acetogens have acetyl-CoA synthetase activity. CO dehydrogenase (EC 1.2.99.2) of the Protein Crystallization. The protein was crystallized under carboxidotrophic bacterium Oligotropha carboxidovorans is a vapor diffusion conditions by using 0.8 M KH2PO4, 0.8 M molybdenum-containing iron-sulfur flavoprotein that cata- NaH2PO4, 2% MPD, and 100 mM Hepes adjusted to pH 7.3. ϩ 3 ϩ Ϫ ϩ ϩ lyzes the oxidation of CO (CO H2O CO2 2e 2H ) Crystals containing cyanide were cocrystallized in the pres- and generates a proton gradient across the cytoplasmic mem- ence of 4 mM potassium cyanide. For synchrotron data brane by channeling the electrons formed via cytochrome b561 collection under low-temperature conditions, 15% glycerol into a CO-insensitive respiratory chain (4). O. carboxidovorans was added to the crystallization buffer. Before measurements, uses the reactions of the Calvin cycle for CO2 fixation (4). In the concentration of glycerol was gradually increased to 25%. the exponential growth phase, cells of O. carboxidovorans have The crystals had an orthorhombic space group P212121 with 87% of CO dehydrogenase associated with the inner aspect of cell dimensions of a ϭ 129.14 Å, b ϭ 140.34 Å, and c ϭ 164.44 the cytoplasmic membrane and 13% located in the cyto- Å and two monomers per asymmetric unit. plasm (6). Structure Determination. With a first data set to 3.0 Å, a CO dehydrogenase is composed of the 88.7-kDa L (809 Patterson search was carried out. The program AMORE (13) residues), 30.2-kDa M (288 residues), and 17.8-kDa S (166 gave a clear solution for a search model constructed from residues) subunits and exists as a dimer of LMS heterotrimers homologous parts of Mop, which comprised a total of about (4). The L subunit carries the molybdenum cofactor, which is 10% of the scattering mass of the asymmetric unit [R factor of a mononuclear complex of Mo and molybdopterin–cytosine 50.8%, with a correlation coefficient (cc) of 29.4 after trans- dinucleotide (MCD) (7). FAD-binding is on the M subunit and Abbreviations: MCD, molybdopterin–cytosine dinucleotide; VAO, The publication costs of this article were defrayed in part by page charge vanillyl–alcohol oxidase. Data deposition: The atomic coordinates have been deposited in the payment. This article must therefore be hereby marked ‘‘advertisement’’ in Protein Data Bank, www.rcsb.org (PDB ID code 1QJ2). accordance with 18 U.S.C. §1734 solely to indicate this fact. †To whom reprint requests should be addressed. E-mail: dobbek@ PNAS is available online at www.pnas.org. biochem.mpg.de. 8884 Downloaded by guest on September 27, 2021 Biochemistry: Dobbek et al. Proc. Natl. Acad. Sci. USA 96 (1999) 8885 lational search for the second monomer, compared with the next highest peak with an R factor of 52.1% and a cc of 24.7 in a resolution range of 14.0–5.0 Å]. Because it was not possible to interpret the calculated density, we initiated multi- wavelength anomalous diffraction data collection at two wave- lengths (remote wavelength and iron K-edge wavelength) by using synchrotron radiation at BW6, DESY, Hamburg. Data sets native1 and native2 were collected on a MAR-Research (Hamburg, Germany) charge-coupled device detector at Ϫ165°C at BW6, DESY and processed by using DENZO and SCALEPACK (14). By using an anomalous difference Fourier map calculated with the phases obtained from the Patterson search solution, the positioning of the four [2Fe–2S] clusters could be achieved. The phasing was carried out by using MLPHARE (15) and SHARP (16) and allowed after solvent flattening and histogram matching the recognition of the molecular boundaries. A phase combination with subsequent solvent flattening and cyclic averaging within the dimer was performed and allowed us to calculate an interpretable elec- FIG. 1. Ribbon representation of the CO dehydrogenase dimer. tron-density map from the two phase sources. For phase The twofold-symmetry axis of the dimer runs vertically in the plane of  modification, the CCP4 (15) package was used. Density inter- the figure. Helices are shown in red, -sheets in blue, and the connecting turns and loops in yellow. The cofactors are depicted in pretation and model building were carried out by using MAIN white. Structure presentations were created by using BOBSCRIPT and (17). The structure was refined by using torsion-angle dynam- RASTER3D. ics, simulated annealing, and maximum likelihood targets for positional refinement as provided by CNS (18). occurs in a redox state that is reduced by two electrons The cyanide-cocrystallized crystal was measured on a compared with the fully oxidized state (19), a tricyclic tetra- MAR-Research (Hamburg, Germany) 300 image plate detec- hydropterin–pyran system. The MCD–molybdenum cofactor ␣ tor at room temperature by using monochromatized CuK- is located in the L subunit, and the two [2Fe–2S] clusters are radiation produced by a conventional rotating anode (Table 1). located in the S subunit (Fig. 2). These prosthetic groups form a pathway for the electrons to the FAD located in the M RESULTS subunit (Fig. 2). The two active sites of the dimer are at a Mo–Mo distance of 52.9 Å with no further cofactors located Overall Structure. CO dehydrogenase is dimeric in solution, between them, indicating two separate catalytic units in CO and two monomers were found in the asymmetric unit. The dehydrogenase. butterfly-shaped dimer (Fig. 1) has overall dimensions of Structure of the Three Subunits. Subunit S represents the ϫ ϫ 2 145 100 70 Å with an accessible surface area of 78,076 Å . iron-sulfur protein of CO dehydrogenase and is clearly divided The two monomers are basically identical, with an rms devi- ␣ into a C- and an N-terminal domain, each binding a [2Fe–2S] ation of 0.30 Å for the C atoms. cluster, which can be distinguished by EPR (8).
Details
-
File Typepdf
-
Upload Time-
-
Content LanguagesEnglish
-
Upload UserAnonymous/Not logged-in
-
File Pages6 Page
-
File Size-