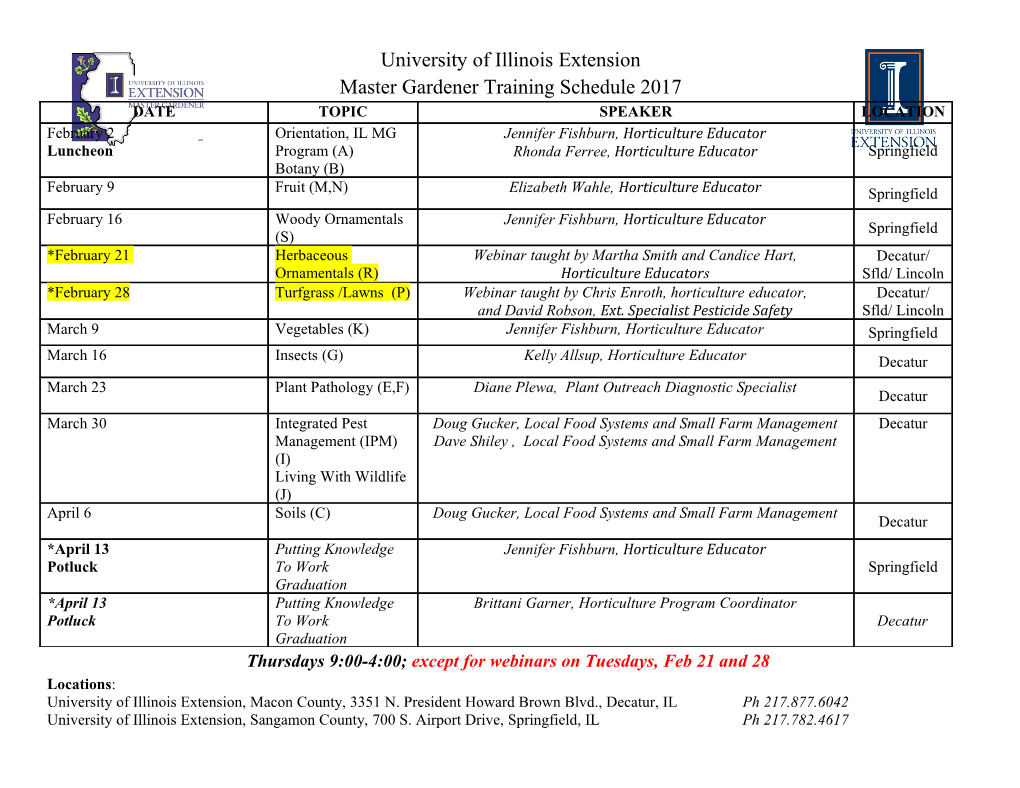
LIFE SUPPORT MATERIALS IN A MANNED SUBMERSIBLE T. C. Wang Harbor Branch Foundation, Inc. RR 1, Box 196 Fort Pierce, Florida 33450 February, 1982 HARBOR BRANCH TECHNICAL REPORT NUMBER 45 LIFE SUPPORT MATERIALS IN A MANNED SUBMERSIBLE T. C. Wang Harbor Branch Foundation, Inc. RR 1, Box 196 Fort Pierce, Florida 33450 February, 1982 HARBOR BRANCH TECHNICAL REPORT NUMBER 45 Table of Contents CARBON DIOXIDE REMOVAL I. Alkali Metal Hydroxide 1. General review 2. Lithium hydroxide 3. Sodalime 4. Baralyme 5. Discussion - water vapor, temperature, humidity, porosity, residence time. II. Alkali Metal Superoxides, Peroxides 1. Sodium or potassium superoxide 2. Alkali metal peroxides III. Regenerative Type of CO2 Absorbent 1. Molecular sieves 2. Metallic oxides A. Magnesium oxide B. Silver oxide 3. Solid amines 4. Liquid amines 5. Carbon dioxide reduction A. Sabatier process B. Bosch reaction 6. Miscellaneous A. Membrane separation B. Freeze-out OXYGEN SOURCES I. High Pressure Gas Storage II. Crogenic Storage III. Chemical Generation 1. Chlorate candle 2. Alkali metal superoxides and peroxides 3. Decomposition of hydrogen peroxide 4. Miscellaneous A. Oxygen generation B. Photosynthesis TRACE CONTAMINANTS REMOVAL I. Contaminant Sorption by Solids 1. Activated charcoal 2. Molecular sieve 3. Silica gel, activated alumina II. Chemical Conversion of Contaminants 1. Hopaclite 2. Purafil GAS PROPERTIES I. Carbon Dioxide II. Nitrogen III. Oxygen IV. Air V. Helium VI. Carbon Monoxide REFERENCES ii CARBON DIOXIDE REMOVAL I. Alkali Metal Hydroxide 1. General review The oxides, hydroxides, peroxides and higher oxide states of the alkali and alkaline earth metals react with carbon dioxide to form carbonate, bicarbonate or hydrates of carbonates and bicarbonates. The theoretical capacities of the various possible CO 2 sorbents is shown in Table 1. Lithium monoxide has a greater CO 2 capacity than any of the other oxides. The use of lithium oxide as a carbon dioxide absorbent has been investigated for short duration space flights. Its dynamic absorption characteristics are not as good as those of lithium hydroxide. Alkali metal hydroxides other than LiOH have a high capacity for CO 2, However, their high degree of hygroscopicity eliminates them from consideration as solid absorbents. Alkaline earth metal oxides such as MgO and CaO absorb carbon dioxide very slowly, while the addition of water vapor and conversion of the oxide to hydroxide increases the rate of absorption. Dedman and Owen (1962), in studying the reaction of CaO with CO 2, showed an initial rapid uptake due to chemisorption, followed by a slower reaction rate­ controlled by the diffusion of CO 2 into the pores of the solid. Comparison of alkaline earth metal hydroxides (see Table 1) shows Mg (OH)2 to have a high capacity for CO 2 on a unit weight basis. Little work has apparently been done on 1 the absorption of CO 2 by magnesium oxide under conditions which are applicable to the removal of C02 from expired gases. The absorption capacity of calcium hydroxide is somewhat less than that of Mg(OH)2' but the literature suggests that the CO 2 absorption rate of hydrated lime is very slow. This slow reaction rate has been circumvented through the use of additives of other hydroxides to the basic lime structure. The addition of 4.5 wt % sodium hydroxide to lime, containing 17.5% water, results in a product which absorbs CO 2 signifi­ cantly more rapidly than Ca{OH)2 alone. This material, commercially known as soda lime, has been used for many years in anesthesiology for the absorption of CO 2 . Another modification of lime is Baralyme, which is a mixture contain­ ing 20 wt % Ba{OH)2 • 8H20 in lime. By far, lithium hydroxide, soda lime and Baralyme are the most effective C02 absorbents in enclosed space. 2 • Lithiurn hydroxide LiOR absorbs CO2 from a gas mixture and in the presence of water vapor. The reaction is given by the following equations: 2LiOH + 2H20 2LiOH •H20 -------- (1) 2LiOH. H + CO Li + 3H ----(2) 20 2 2C03 20 The reaction of C02 with LiOH requires the presence of water in an amount sufficient to produce LiOH • H20 prior to or simultaneously with the CO2 reaction (Williams, 1969; Wang, 1979). The properties of LiOH as a CO 2 absorbent are listed below: 2 Properties of LiaR Chemical formula LiOH Molecular wt. 23.95 Melting point 862°F Density of crystals 0.0918 Ib/in3 Bulk density of granular LiOH 0.0145-0.0162 Ib/in3 Theoretical CO 2 absorption capacity 0.919 Ib C02/1b LiaR Heat of absorption (H20 gas) 875 Btu/lb C02 Water of reaction 0.409 Ib/lb CO 2 or 0.375 Ibjlb LiOR The dynamic removal efficiency, which expresses the reduction in CO 2 concentration across the bed, varies with time in the operation of LiOH as the absorbent is used up. The theoretical capacity of LiOH for CO2 absorption can not be achieved in an atmosphere control system because of the bed dynamics, characteristics, canister dimensions, air superficial velocity and the process air inlet temperature. The LiOR particle size is generally kept between 4 to 8 mesh size for high utilization efficiencies on low bed pressure drops. Normally, no channeling occurs with this particle size at gas velocities up to 1.0 ft/sec (Bentz, 1976). The CO2 absorption capacity of LiOE varies with various temperatures and humidities (Wang, 1975). The chemical reaction associated with water production and the rate of dehydration affect the C02 absorption capacity (see discussion) . 3 3. Sodasorb (Soda Lime) Sodasorb consists essentially of hydrated lime [Ca(OH)2] , sodium hydroxide (NaOH), potassium hydroxide (KOH) , 14 to 19 percent moisture content, and some inert. It is in the form of granules and contains an indicator which changes from colorless or white to blue when the NaOH has reacted to form Na2C03' The chemical reaction of CO 2 with Sodasorb is as follows: CO 2 + H20 + H2Co 3 ... (3) 2 H2C03 + 2 NaOH + 2 KOH + Na2Co3 + K2C0 3 + 4 H20 .•. (4) Na2C03 + K2C03 + 2 Ca(OH)2 + 2 CaC03 + 2 NaOH + 2 KOH ... (5) The water is added as free moisture in the manufacturing process and is necessary for efficient removal of CO 2, Sig­ nificant water is lost through evaporation if properly sealed storage is not maintained and the C02 absorption capacity will be decreased. The theoretical C02 absorption capacity and physical properties of Sodasorb are shown in Tables 1 and 3. 4. Baralyme Baralyme is a mixture of barium hydroxide octalhydrate [Ba(OH)2 • 8H20] , calcium hydroxide [Ca(OH)2] and potassium hydroxide (KOH). The eight waters of crystallization present in the Ba(OH)2 serve to fuse the mixture into a homogeneous mass which will hold its shape and form under varying conditions of heat and moisture. The water is used as shown in the chemical equations: 4 Ba(OH)2° S H2O + CO 2 -+ BaC03 + 9 H20 ... (6) 9 H2 0 + 9 CO 2 -+ 9 H2C03 ......... (7) 9 H2 C03 + 9 Ca(OH)2 -+ 9 CaC03 + IS H20 ... (S) 2 KOH + H2C03 -+ K2C03 + 2 H20 ... (9) Ca(OH)2 + K2C03 -+ CaC03 + 2 KOH ... (lO) When temperature is above 150°F, water has a tendency to liberate from Baralyme granules and CO 2 absorption capacity will be decreased. Tables 1 and 3 show the properties, characteristics and CO 2 absorption capacity of Baralyme. 5. Discussion Lithium hydroxide, Sodasorb and Baralyme are the most widely used absorbents used for CO2 removal. Experience has shown that the CO 2 removal is related to the porosity, granule size, surface area, moisture content, scrubber design and other environmental conditions such as temperature, humidity, pressure, etc. (Boryta, 1967; Cook, 1972; Mi Ll.er , 1979; Wang, 1975; Williams, 1969). Insufficient water vapor in the air stream allows only partial CO 2 reactions as shown in the chemical equations, while an excessive amount of water vapor forms a water film barrier around the absorbent granules also resulting in an incomplete reaction between CO 2 and absorbents (Williams, 1969; v7ang, 1979, 19S0). Higher temperature normally favors higher reaction rate and therefore has a higher water production. Too high a temperature in the chemical reaction produces excess amounts of water that could decrease the available surface area for CO 2 reaction. 5 When this occurs, the CO 2 absorption capacity of absorbents decreases. For a given CO 2 content in the alr stream at any given temperature, there is a corresponding humidity of the feed stream which results in maximum C02 absorption efficiency. When the relative humidity in the air stream is below 14%, anhydrous LioH has a higher CO 2 absorption capacity at higher temperatures. However, above 40% relative humidity, lower temperatures are favored as shown in Figure 1 (Wang, 1979). The CO2 absorption capacity of Sodasorb and Baralyme at various temperatures and humidities are shown in Figures 3-5 (Wang, 1980). When temperature is less than 65°F, water content in the air stream does not significantly affect CO 2 absorption capacity. When temperature is greater than 65°F, the CO2 absorption capacity of both Sodasorb and Baralyme are increased with increased temperature (Wang, 1980). Porosity with surface area in the granules also controls the CO 2-absorbent reaction rate (Wang, 1978, 1980). The more porous granules provide more available surface area for the CO2 reaction with the absorbents. Water and C02 can more easily diffuse into the inner parts of the granules which R results in a higher CO 2 absorption capacity. Sodasorb Hp is about two times more porous than Sodasorb as shown in Table 3. The CO2 absorption capacity of Sodasorb HP was found to be 2.5 times more efficient than Sodasorb granules (Wang, 1978, 1980) .
Details
-
File Typepdf
-
Upload Time-
-
Content LanguagesEnglish
-
Upload UserAnonymous/Not logged-in
-
File Pages63 Page
-
File Size-