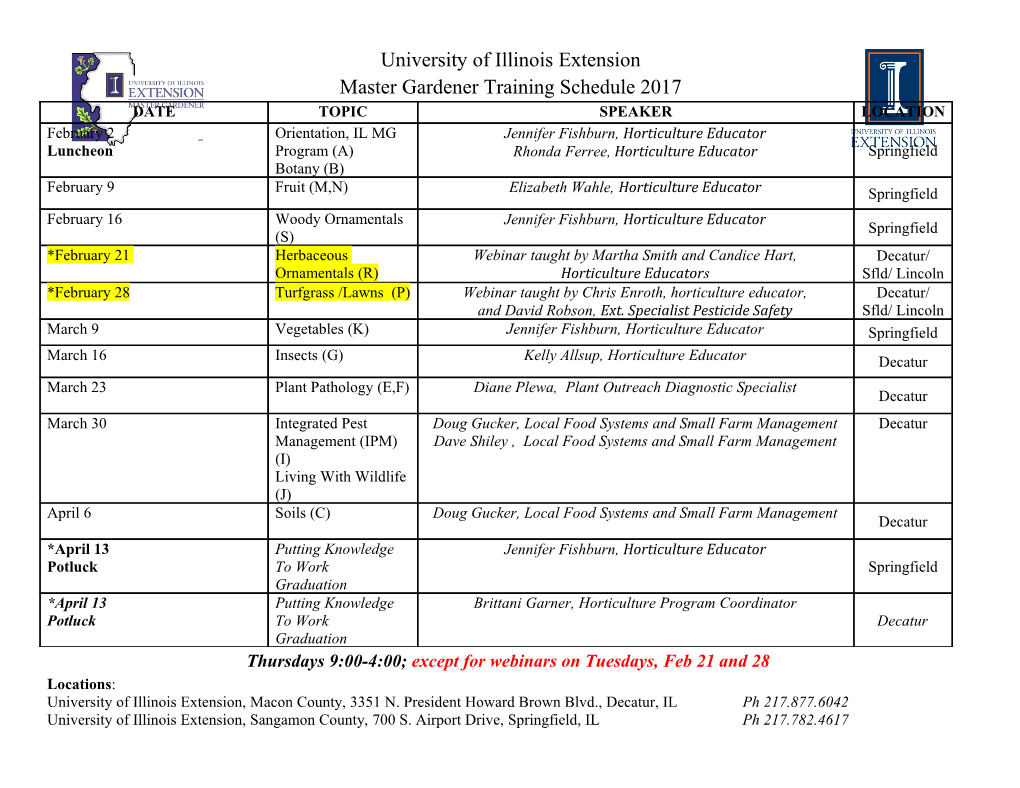
Modelling tokamak power exhaust and scrape-off-layer thermal transport in high-power fusion devices MICHAEL ROBERT KNOX WIGRAM Doctor of Philosophy University of York Physics December 2019 2 3 Abstract Managing the steady-state power loading onto the divertor target plates remains a major unresolved challenge facing tokamak fusion energy, that will be crucial for the success of the next generation of high-power reactor-level devices. This thesis will tackle two topics within this wide research area: assessing the performance of advanced divertor geometries in the context of the ARC reactor concept, and studying the impact of `nonlocal' thermal transport on modelling predictions for the ITER tokamak SOL. Numerical simulations are performed using UEDGE for the Super-X divertor (SXD) and X-point target divertor (XPTD) configurations proposed for the ARC reactor design. The SXD, combined with 0.5% fixed-fraction neon impurity concentration, produced passively stable, detached divertor regimes for power exhausts in the range of 80-108 MW. The XPTD configuration is found to reduce the strike-point temperature by a factor of ∼10 compared to the SXD for small X-point radial separations (∼1.4λqjj). Even greater potential reductions are identified for separations of ≤1λqjj. Raising the separatrix density by a factor 1.5, stable detached divertor solutions were obtained that fully accommodate the ARC exhaust power without impurity seeding. In the presence of steep temperature gradients, classical local transport theory breaks down, and thermal transport becomes nonlocal, depending on conditions in distant regions of the plasma. An advanced nonlocal thermal transport model is implemented into the `SD1D' complex SOL code to create `SD1D-nonlocal', and applied to study typical ITER steady- state conditions. Results suggest that nonlocal transport effects will have importance for the ITER SOL, with discrepancies observed between nonlocal/local transport model predictions in low-density scenarios. Heat flux models employing global flux limiters are shown to be inadequate to capture the spatially/temporally changing SOL conditions. An analysis of SOL collisionality and nonlocality suggests nonlocal effects will be significant for future devices such as DEMO and ARC as well. Contents Abstract 3 List of Tables 8 List of Figures 9 Acknowledgements 13 Declaration 15 1 Introduction 17 1.1 Nuclear Fusion . 17 1.2 Magnetic Confinement Fusion . 20 1.3 High-power reactors . 22 1.3.1 Current/future devices . 22 1.3.2 Exhaust and modelling challenges facing high-power reactors . 25 1.4 Thesis outline . 27 2 The tokamak SOL and divertor 29 2.1 The scrape-off-layer plasma . 29 2.2 The divertor SOL . 30 2.2.1 Plasma-surface interactions . 32 2.2.2 Attached plasma regimes - sheath and conduction limited SOL . 37 2.2.3 Detachment . 38 2.2.4 Cross-field transport . 40 2.2.5 Divertor geometry . 41 2.3 The ITER divertor . 42 4 CONTENTS 5 2.4 Advanced divertors . 43 2.4.1 Double-null . 44 2.4.2 X-divertor . 45 2.4.3 Super-X Divertor (SXD) . 45 2.4.4 Snowflake divertor . 46 2.4.5 X-Point Target Divertor (XPTD) . 47 3 Modelling the tokamak SOL 48 3.1 SOL fluid description/Braginskii equations . 48 3.1.1 Particle conservation - continuity equation . 49 3.1.2 Momentum conservation equation . 49 3.1.3 Energy conservation equation . 50 3.1.4 Braginskii equations . 51 3.2 1D SOL modelling - SD1D . 53 3.3 2D SOL modelling - UEDGE . 58 3.4 Numerical methods . 63 3.4.1 Backward differentiation formulae . 63 3.4.2 Newton-Krylov method . 64 3.4.3 SD1D - finite differencing methods . 66 3.4.4 UEDGE - finite volume methods . 68 4 ARC reactor 70 4.1 Compact fusion reactor concept and ARC design . 70 4.2 ARC divertor design . 77 5 ARC Divertor Modelling 81 5.1 UEDGE ARC SOL physics model . 81 5.2 ARC Super-X Divertor . 86 5.2.1 Without impurity seeding . 86 5.2.2 With 0.5% Neon impurity seeding . 88 5.2.3 Convergence testing . 90 5.3 ARC X-Point Target Divertor . 91 5.4 Model sensitivity studies for the XPTD . 95 CONTENTS 6 5.4.1 Upstream density . 96 5.4.2 Leg radial transport coefficients D and vconv . 98 5.4.3 HFS:LFS power split . 102 5.5 Discussion . 105 5.5.1 Long-legged divertor performance . 105 5.5.2 SXD . 105 5.5.3 XPTD . 106 5.5.4 Model improvements and opportunities for further study . 107 6 Nonlocal thermal transport in the SOL 113 6.1 Kinetic nonlocal thermal transport effects in plasmas . 114 6.1.1 Local thermal transport - Spitzer-H¨arm . 114 6.1.2 Low collisionality and nonlocal thermal transport . 115 6.2 Capturing nonlocal effects in SOL modelling . 117 6.2.1 Flux limiters . 118 6.2.2 Convolution Models . 120 6.2.3 Reduced-kinetic models . 121 6.3 The Ji-Held nonlocal model . 122 6.4 Implementation of `SD1D-nonlocal' code . 125 7 SD1D-nonlocal ITER simulations 129 7.1 1D ITER-like tokamak . 129 7.1.1 Convergence testing . 132 7.2 Varied ITER SOL collisionality . 133 7.3 Predicting SOL nonlocality . 135 7.3.1 Assessing nonlocality using local temperature scalelength . 135 7.3.2 Assessing nonlocality using connection length . 137 7.4 Impurity seeding . 141 7.5 Discussion . 145 8 Summary and Conclusions 149 8.1 ARC divertor modelling . 149 8.2 Nonlocal thermal transport for ITER . 151 CONTENTS 7 A Effect of sheath boundary condition on LT /λe metric 153 List of References 154 List of Tables 4.1 ARC design parameters . 73 4.2 Inter-machine comparison of divertor heat-loading metrics. 77 ∗ 7.1 SOL parameter values and calculated νSOL for various fusion devices . 138 8 List of Figures 1.1 Binding energy of atomic nuclei . 18 1.2 Cross-sections of fusion reactions . 19 1.3 Plasma gyromotion and tokamak schematic . 21 1.4 Cross-section of tokamak limiter and divertor configurations. 22 1.5 JET and ITER tokamaks. 23 1.6 DEMO and ARC tokamaks. 24 2.1 Schematic of divertor magnetic geometry . 31 2.2 Illustration of the sheath and presheath effects in a plasma . 33 2.3 SOL detachment conditions at the divertor target . 39 2.4 Vertical and horizontal target plate tilting . 42 2.5 Open and closed divertor configurations . 42 2.6 CAD diagram of the ITER divertor . 43 2.7 Illustration of double-null divertor configuration . 44 2.8 Illustration of XD and SXD configurations . 45 2.9 Snowflake divertor configuration . 46 2.10 X-Point Target Divertor configuration . 47 3.1 Illustration of SD1D simulation domain . 57 3.2 Diagram of the SD1D simulation grid . 67 3.3 Example UEDGE simulation grid for DIII-D . 68 4.1 3D design projection of the ARC reactor. 71 4.2 ARC core profiles for Te and ne. ......................... 75 4.3 Schematic diagram of the ARC X-point target divertor. 79 9 LIST OF FIGURES 10 5.1 Schematic diagram of UEDGE ARC SOL/divertor grids for the SXD and XPTD configurations . 82 5.2 Outer midplane profiles for D, χi;e and vconv defined for the UEDGE transport model . 84 5.3 ARC model outer midplane profiles for n, Te and Ti, as well as qjj profile at the primary X-point location . 84 5.4 2D Te plots for ARC SXD steady-state solutions without impurity seeding and with 0.5% neon impurity fraction . 87 5.5 SXD power scan results showing peak outer plate Te (eV) against exhaust power PSOL (MW) for 0% and 0.5% neon fraction . 88 5.6 2D plots of Te (with peak power flux densities to the boundaries) and neon impurity radiation emissivity for detached SXD solution . 89 5.7 Upswing and downswing power scans for the SXD and XPTD configurations for varying X-point separations and measured λqjj against X-point radial sep- aration for each case . 92 5.8 Upswing and downswing power scans for the SXD and XPTD configurations for varying X-point separations and with new measured λqjj with adjusted transport coefficients . 92 5.9 Downswing/upsing power scan detachment thresholds (MW) and peak plate Te (eV) at PSOL = 105 MW against XPTD X-point separation . 93 5.10 Plots.
Details
-
File Typepdf
-
Upload Time-
-
Content LanguagesEnglish
-
Upload UserAnonymous/Not logged-in
-
File Pages164 Page
-
File Size-