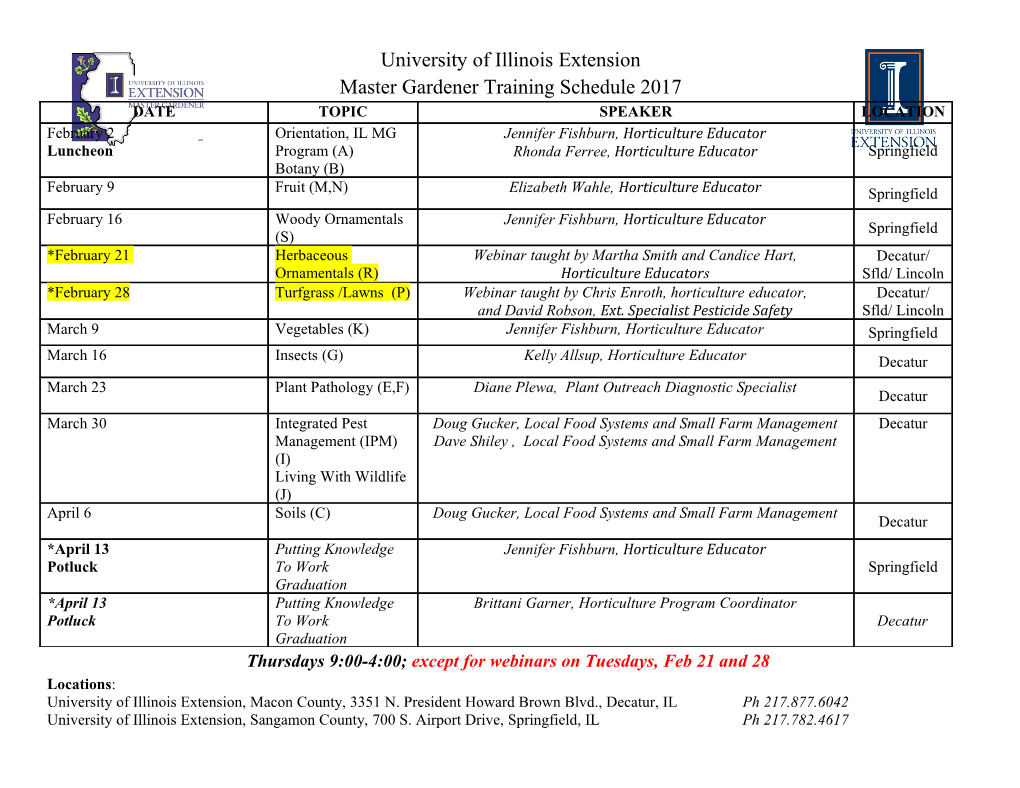
Chapter 6 Theory of ENSO Sensitivity to Climate All perception of truth is the detection of an analogy. Thoreau, Journal, 1851 In this chapter we examine the role of the background state in the dynamics of ENSO, by examining the processes that drive sea surface temperature changes in the equatorial Pacific. We first review the primary modes that contribute to interannual oscillations, and examine previous model results regarding the sensitivity of ENSO to the climatology. We then investigate the influence of nonlinearity and the climatology on these modes and propose a way of distinguishing between them based on the relative phasing of terms in the SST tendency equation. Phasor diagrams prove useful for clarifying the individual roles of the tendency terms and their sensitivities to climate change. 6.1 Literature review 6.1.1 Prototype modes Comprehensive reviews of ENSO theory are given by Neelin et al. (1998) and Philander (1999). In this section we shall focus only on the aspects most relevant to understanding the ENSO sensitivity to the tropical climatology. Two basic types of coupled ocean-atmosphere modes appear relevant to the observed ENSO: a “delayed-oscillator” or “recharge” mode (Fig. 6.1) which depends on slow oceanic adjustment to remote wind forcing (Wyrtki, 1985; Schopf and Suarez, 1988; Suarez and Schopf, 1988; Battisti and Hirst, 1989; Schneider et al., 1995; Jin, 1996; Li, 1997; Jin, 1997), and “SST” modes (Fig. 6.2) that depend more on local wind forcing, anomalous zonal currents, and anomalous upwelling (Zebiak, 1985; Hirst, 1986; Neelin, 1991; Hao et al., 1993; Jin and Neelin, 1993a,b; Dijkstra and Neelin, 1999). ENSO in the real world appears to be a hybrid mode, with ocean adjustment setting the time scale and the SST mode setting the spatial structure and propagation 175 Chapter 6: Theory of ENSO Sensitivity to Climate 176 ×haÐÐÓÛ ¼ deeÔ Ü ×haÐÐÓÛ Figure 6.1: View of the recharge/delayed oscillator mode from above. An equatorially- confined westerly stress anomaly induces a rapid deepening of the thermocline in the equa- torial eastern Pacific, which warms the surface there via mean upwelling and enhances the westerly stress anomaly. Meanwhile, strong cyclonic stress curl off-equator induces a broad shoaling of the thermocline in the western Pacific and slowly discharges warm water from the equatorial zone. Narrow western boundary currents return some warm water to the equator but not enough to compensate for the discharge in the interior. As the equato- rial thermocline shoals, the warm event decays as the remaining wind forcing continues discharging water from the equator. The system is then carried into a cold event by an anomalously shallow equatorial thermocline and associated westward current anomalies, and the cycle repeats with opposite sign. Chapter 6: Theory of ENSO Sensitivity to Climate 177 ¼ Ü ¼ ÛaÖÑ Ù ¼ Û ¼ h Figure 6.2: Structure of the SST mode, represented as a vertical section through the oceanic active layer and lower troposphere at the equator. A warm anomaly induces anomalous westerly wind stress to the west, which induces downwelling anomalies local to the stress and westerly current anomalies slightly west of the stress. The anomalous currents thus favor westward propagation by acting on the mean upward and westward thermal gradients to induce warming to the west of the warm anomaly. In the fast-wave limit, the thermocline is anomalously deep to the east of the stress anomaly, which favors eastward propagation of the SST anomaly if the mean upwelling is strong enough. of SST anomalies (Jin and Neelin, 1993a). If the modal period is long enough (a few years), as appears to be the case for the observed ENSO, individual Kelvin and Rossby wavefronts may not be apparent; hence the relevance of a “fast-wave limit” for the SST mode in which the subsurface ocean is assumed to adjust instantaneously to the wind stress (Neelin, 1991). Variability at shorter periods (a year or less), on the other hand, can evolve as a coupled Rossby mode that depends primarily on zonal advection at the equator and resonant interactions with the eastern and western boundaries (Hirst, 1986; Mantua and Battisti, 1995); such modes have been found to play a role in some models (e.g. that of Zebiak and Cane, 1987). The zonal propagation of SST anomalies in the fast-wave limit is set by a competi- tion between “thermocline feedbacks” and “local” or “advective” feedbacks (Hirst, 1985, 1986, 1988; Hao et al., 1993; Jin and Neelin, 1993a; An and Jin, 2000, 2001). Thermo- cline feedbacks are associated with the equatorial thermocline slope, which responds fairly rapidly to changes in zonal wind stress; a westerly stress anomaly induces a deepening of the thermocline in the east, which warms the surface in the east through mean upwelling and drags the stress anomaly eastward. The local advective feedbacks are associated with the anomalous zonal currents and upwelling, which respond locally to stress anomalies that are generally shifted west of the SST anomalies; a warm SST anomaly induces westerlies to the west, which induce local downwelling and eastward currents that drag the SST anomaly westward. Thus thermocline and local advective feedbacks tend to favor eastward and westward propagation, respectively. If both feedbacks are present, the propagation Chapter 6: Theory of ENSO Sensitivity to Climate 178 can be slowed or halted; in the latter case, a standing SST oscillation may arise if oceanic adjustment is delayed. The stability and period of the delayed/recharge oscillator likewise results from a com- petition of feedbacks (Battisti and Hirst, 1989; Jin, 1997; An and Jin, 2001). The first is local growth in the equatorial eastern Pacific, which is accomplished by a basinwide positive feedback: during El Ni˜no, the trade winds slacken and deepen the thermocline in the east, which inhibits cooling of the surface in the east, weakens the zonal SST gradient, and thereby further weakens the trades. The second process is a delayed negative feedback that results from slow adjustment of the thermocline. In response to meridionally-confined wind anomalies such as occur during ENSO, geostrophic divergence in the interior and western boundary currents discharge (during El Ni˜no) or recharge (during La Ni˜na) warm surface waters at the equator, which raises or lowers the thermocline in opposition to the eastern Pacific anomaly generated by the zonal slope of the thermocline. Eventually, this slow adjustment affects the surface waters sufficiently to bring about a reversal of the event. Zonal currents, which are linked geostrophically to the zonal-mean thermocline depth at the equator, also contribute to the decay by advecting cold water westward (fol- lowing an El Ni˜no) or warm water eastward (following La Ni˜na). The combination of rapid adjustment of the equatorial thermocline slope, and delayed adjustment of the equatorial zonal-mean thermocline induced by off-equatorial ocean memory, are thus essential for this mode. The period and growth rate of this delayed/recharge oscillator are surprisingly complex functions of the adjustment time, the strength of the delayed feedbacks, and the strength of local growth processes in the eastern equatorial Pacific (Battisti and Hirst, 1989). These “prototype modes” can be hard to characterize simply by looking at SST; their similar spatial structures can belie their internal mechanisms and thus their sensitivities to externally-imposed changes. The best way to distinguish them is to examine the balances and relative phasings of the processes which affect SST. We shall develop a framework for this in Sections 6.2 and 6.3. 6.1.2 Influence of the background state On equatorial waves From the shallow-water equations (4.1)–(4.3) it is evident that the depth of the ther- mocline affects the speed and amplitude of equatorial waves. Using an intermediate model, Zebiak and Cane (1987) found that a shoaling of the zonal-mean thermocline, by ampli- fying the thermocline-slope response to wind stress and delaying the adjustment of the zonal-mean thermocline to changes in wind stress, tended to increase the amplitude and period of ENSO; Dewitte (2000) found similar results. Other authors have expanded on how the structure of the climatology can influence the amplitude, propagation, and disper- sion of Kelvin and Rossby waves in a multilevel ocean (Busalacchi and Cane, 1988; Yang, 1991; Yang and Yu, 1992; Yang and O’Brien, 1993). The background state can also affect the propagation of equatorial waves in the at- mosphere. Wang and Xie (1996) found that increased mean vertical westerly wind shear tended to weaken Rossby waves in the lower troposphere, with little impact on Kelvin Chapter 6: Theory of ENSO Sensitivity to Climate 179 waves. Since it is the Rossby component that conveys the westerly stress response west- ward from regions of anomalous heating, it is conceivable that a long-term change in the strength of the Walker Cell could affect the structure of the wind stress response to SST anomalies. The mean vertical wind shear can also affect the surface stress profile of intrasea- sonal convective disturbances like the MJO, which appear to be important for modifying ENSO evolution. Takayabu et al. (1999), for example, argued that background westerly shear contributed to the rapid demise of the 1997–98 El Ni˜no: westerly shear altered an MJO disturbance so that it produced predominantly easterly stress anomalies, which in May 1998 reinitiated upwelling on an unusually strong subsurface temperature gradient in the eastern Pacific. In conceptual models Results from simple conceptual models provide some insight into the climate sensitiv- ities of ENSO. Battisti and Hirst (1989) analyzed a simple delayed-oscillator model, and found that a strengthening of local growth mechanisms in the east Pacific, or an increase in the delay time for negative feedbacks, favored longer-period and faster-growing oscilla- tions.
Details
-
File Typepdf
-
Upload Time-
-
Content LanguagesEnglish
-
Upload UserAnonymous/Not logged-in
-
File Pages18 Page
-
File Size-