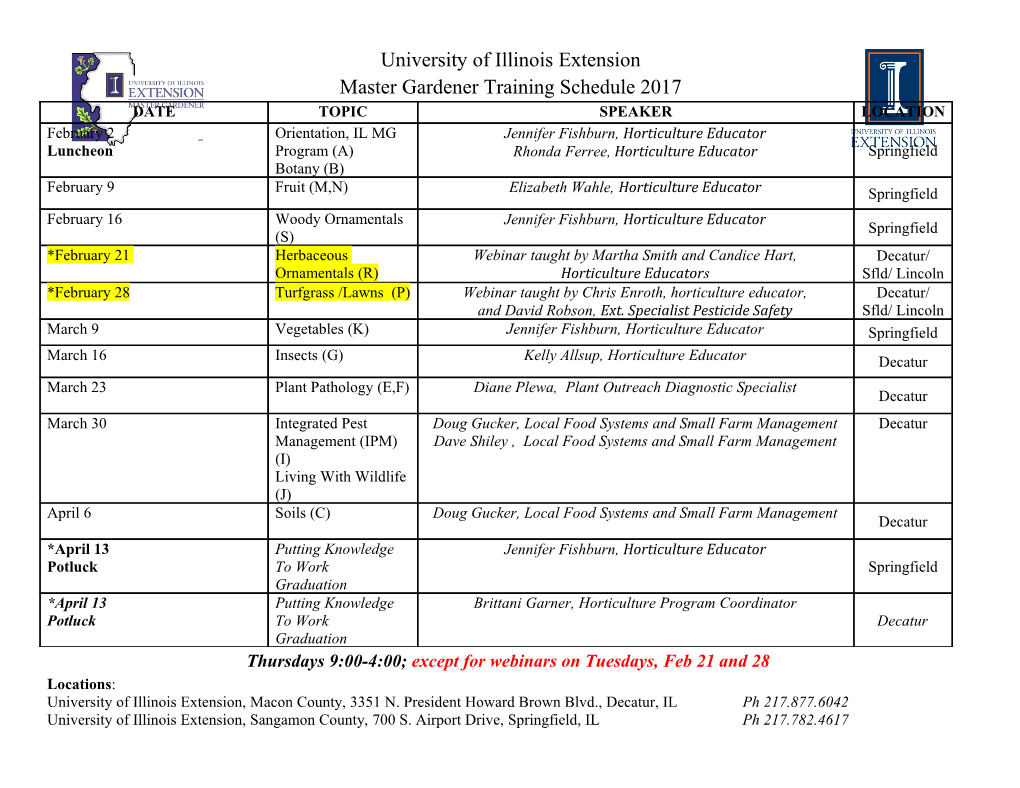
ELECTRONIC TRANSPORT AT SEMICONDUCTOR AND PEROVSKITE OXIDE INTERFACES by NICHOLAS JAMES GOBLE Submitted in partial fulfillment of the requirements for the degree of Doctor of Philosophy Department of Physics CASE WESTERN RESERVE UNIVERSITY May, 2016 CASE WESTERN RESERVE UNIVERSITY SCHOOL OF GRADUATE STUDIES We hereby approve the thesis/dissertation of Nicholas James Goble candidate for the degree of Doctor of Philosophy*. Committee Chair Xuan P.A. Gao Committee Member Harsh Mathur Committee Member Kathleen Kash Committee Member Alp Sehirlioglu Date of Defense December 11, 2015 *We also certify that written approval has been obtained for any proprietary material contained therein. ii To my wife, Mary. Your love and support has made this all possible. iii Table of Contents Chapter 1 Introduction .................................................................................................... 1 1.1 Introducing quantum mechanics to electronic transport ........................................... 4 1.1 Two-dimensional electronic systems ........................................................................ 5 1.2 Materials of interest .................................................................................................. 9 1.2a GaAs/AlxGa1-xAs quantum wells ....................................................................... 10 1.2b LaAlO3/SrTiO3 heterointerfaces ....................................................................... 16 1.2c γ-Al2O3/SrTiO3 heterointerfaces ........................................................................ 19 1.3 Structural phase transition in SrTiO3 ...................................................................... 20 1.3a Structural phase transitions in two dimensions ................................................. 21 1.3b Cubic-to-tetragonal phase transition in SrTiO3 ................................................. 23 Chapter 2 Samples and experimental setup ................................................................. 26 2.1 GaAs/AlxGa1-xAs samples ....................................................................................... 26 2.2 Perovskite oxide samples ........................................................................................ 28 2.2a γ-Al2O3/SrTiO3 .................................................................................................. 29 2.2b LaAlO3/SrTiO3 .................................................................................................. 29 2.3 Micron-scale fabrication of LaAlO3/SrTiO3 ........................................................... 30 2.4 Tetragonal domain imaging of LaAlO3/SrTiO3 ...................................................... 31 2.5 Low-temperature/low-noise electrical transport measurements ............................. 32 Chapter 3: Short-range scattering on two-dimensional holes in quantum wells ...... 36 3.1 Scattering mechanisms and the nonmonotonic resistance peak ............................. 36 3.2 The effect of quantum wall barrier height on electronic transport ......................... 38 3.3 Longitudinal resistance in the Bloch-Gruneisen regime ......................................... 40 iv 3.4. Characteristic temperature in response to disorder ................................................ 44 3.5 Universality of the electronic contribution and its implications ............................. 45 Chapter 4 Metal-insulator transition in perovskite oxide heterointerfaces ............. 51 4.1 Strontium titanate in an electric field ...................................................................... 52 4.2 Gating effects of STO ............................................................................................. 55 4.3 Immobile charge carriers ........................................................................................ 57 4.4 Mott insulating behavior ......................................................................................... 59 Chapter 5 Anisotropic electronic transport in an oxide heterointerface ................... 62 5.1 Cubic-to-tetragonal phase transition in LaAlO3/SrTiO3 ......................................... 63 5.2 Phase transitions affecting electronic transport ...................................................... 67 5.3 Anisotropic resistance due to tetragonal domain walls ........................................... 69 5.4 Electrostatic simulation of domain walls ................................................................ 72 Chapter 6 Summaries and perspectives ........................................................................ 75 Bibliography .................................................................................................................... 78 v List of Tables Table 5.1: Collection of patterned and measured samples. All seven samples studied were grown using pulsed laser deposition. A LAO thickness of 10 u.c. provided reliable interfacial conduction in all growth conditions, though a sample with 8 u.c. LAO thickness was studied as well, providing comparable results. Samples grown at a lower O2 partial pressure showed substantially higher carrier densities due to the increase in oxygen vacancies in the STO crystal. Sample 10a.615 was patterned with eight contacts, allowing for multiple measurement configurations across the same domain wall. ......................... 66 vi List of Figures Figure 1.1. (a) Energy band structure for two separate materials with different energy gaps. At room temperature, GaAs has a direct band gap of 1.42 eV, while AlxGa1-xAs has a direct band gap of 1.42 + 1.25x eV for x < 0.45. (b) Energy band bending of GaAs/AlGaAs heterointerface. To reach an equilibrium of the Fermi level, energy bands are bent, forming a triangular quantum well on the GaAs side of the interface. (c) Density of states of a two- dimensional electron gas with only one occupied subband, where EF < E2. Inset is the energy levels of a triangular quantum well. ........................................................................ 8 Figure 1.2. Zincblende crystal structure of GaAs and AlGaAs. For GaAs, the color of the spheres represents atoms of different types, though the assignment is arbitrary. Without purple spheres, the structure is a basic face-centered cubic (FCC) unit cell. A zincblende structure can be created by overlaying two FCC unit cells (lattice parameter a) of different atoms offset by (a4, a4, a4). ............................................................................................. 11 Figure 1.3. (a) Perovskite unit cell of strontium titanate. An octahedral of oxygen atoms occupy the face centers of the cubic crystal formed by strontium atoms. (b) Cross sectional slice of a 4-unit cell (u.c.) lanthanum aluminate/strontium titanate heterointerface. The junction must be TiO2-terminated SrTiO3. ........................................................................ 17 Figure 1.4. Lattice spacing for a two-dimensional cubic lattice as it transitions to tetragonal. At temperature T > Tc, the crystal structure is square (a) with a lattice constant of a0. As the temperature is lowered, buckling occurs (b) and the lattice spacing is reduced, a < a0. The temperature is lowered past the critical temperature, T < Tc, below which the crystal transitions into a triangular structure with a spacing of a1. .............................................. 21 vii Figure 1.5. (a) Two-dimensional oblique phase with domain D1. (b) Two-dimensional oblique phase with domain D2. (c) Tiling rule for domains D1 and D2 in the oblique crystal phase. The intersection of crystal domains form a domain wall based on tiling rules. (d) A striped phase of domains D1 and D2 forms, resembling a buckling phase. ....................... 22 Figure 1.6. (a) Cubic, perovskite structure of strontium titanate at 300K. Blue, green, and red spheres represent strontium, oxygen, and titanium, respectively. Oxygen atoms form an octahedra. (b) In the tetragonal phase, the oxygen octahedra rotates with an angle, θ, and the axis, c, orthogonal to the octahedral rotation extends slightly. ............................ 24 Figure 2.1. (a) Three-dimensional schematic of a typical GaAs quantum well sample. InZn contacts are placed in a square Van der Pauw pattern and annealed deep below the surface. (b) Layer structure for GaAs quantum wells used. Note the InZn contact diffuses through the upper GaAs layers to make a solid electrical connection to the two-dimensional electron gas. .................................................................................................................................... 26 Figure 2.2. (a) Three-dimensional schematic of a typical perovskite oxide heterointerface sample. The schematic is the same for either LaAlO3/SrTiO3 or gamma-alumina/SrTiO3. Aluminum contacts are wire bonded in a square Van der Pauw pattern, which pierce through the upper oxide to contact the interface. (b) Layer structure for GaAs quantum wells used. Note the InZn contact diffuses through the upper GaAs layers to make a solid electrical connection to the two-dimensional electron gas. .............................................. 28 Figure 2.3. Sample reduction process. (a) As-is LaAlO3/SrTiO3 crystal with a conductive two-dimensional electron gas at the interface. After photolithography, a (b) Van der Pauw photoresist mask remains adhered to the LaAlO3 surface. The unmasked LaAlO3 and viii underlying SrTiO3 are etched 5-7 nm deep with hydrofluoric acid, removing the two- dimensional conductive interface everywhere
Details
-
File Typepdf
-
Upload Time-
-
Content LanguagesEnglish
-
Upload UserAnonymous/Not logged-in
-
File Pages107 Page
-
File Size-