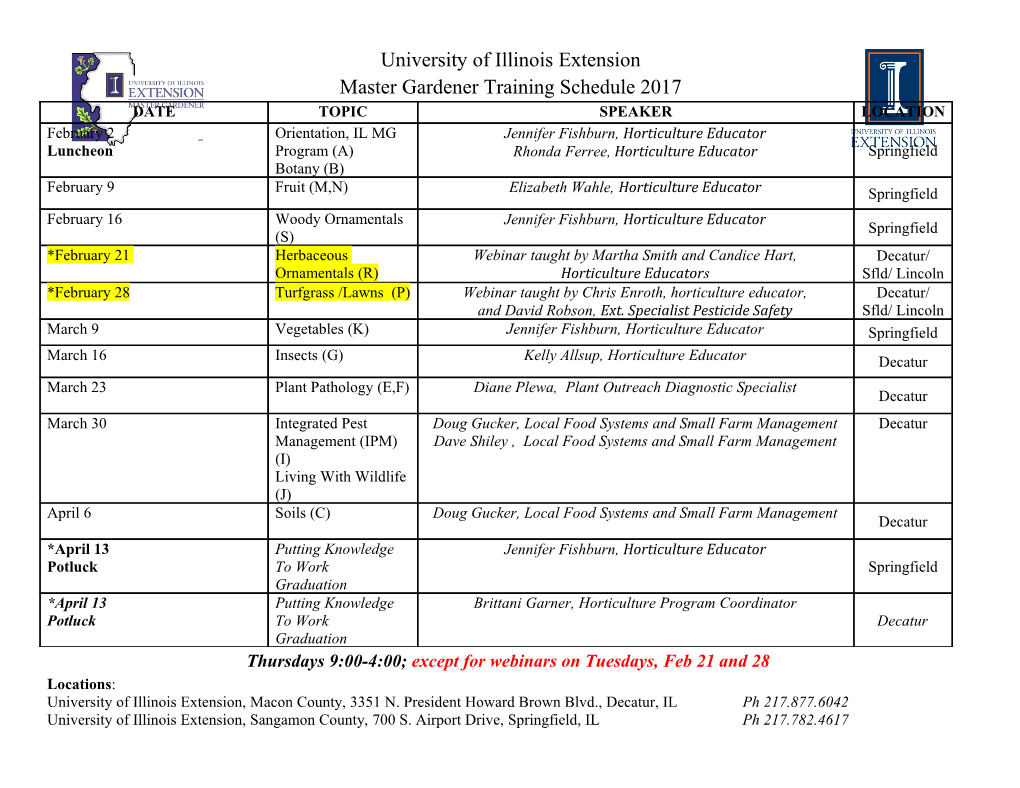
Acta Materialia 55 (2007) 2397–2406 www.actamat-journals.com Five branching growth patterns in the cubic crystal system: A direct observation of cuprous oxide microcrystals Jiasheng Xu, Dongfeng Xue * State Key Laboratory of Fine Chemicals, Department of Materials Science and Chemical Engineering, School of Chemical Engineering, Dalian University of Technology, 158 Zhongshan Road, Dalian 116012, China Received 29 September 2006; received in revised form 13 November 2006; accepted 17 November 2006 Available online 1 February 2007 Abstract Cuprous oxide (Cu2O) is selected in this paper to elucidate the shape evolution in the cubic crystal system. A wide range of novel cuprous oxide microcrystals (based on the five branching growth patterns) has been prepared through an ethylenediaminetetraacetic acid tetrasodium salt dihydrate (EDTA) reduction route by employing the EDTA molecule as both chelating reagent and reductant. The mor- phology of these microcrystals has a strong dependence on the reaction conditions, which implies vast possibilities of designing new crys- tal morphologies and may result in the development of powerful and economical design strategies to enable future progress. Ó 2006 Acta Materialia Inc. Published by Elsevier Ltd. All rights reserved. Keywords: Crystal morphology; Branching growth; Cubic crystal system; Cuprous oxide 1. Introduction patterns whose artistic value alone is worth understanding [27]. Crystal growth is a universal phenomenon in the field of A baseline for crystal morphology is the equilibrium materials science and technology. Knowledge of the funda- shape that results from minimizing the anisotropic surface mentals of crystal growth is due entirely to the morpholog- free energy of a crystal under the constraint of constant ical studies of naturally occurring crystals, which slowly led volume. As is well known, the equilibrium shape that cor- to the understanding of the atomistic process of crystal responds to a given c-plot is given by the Wulff construc- growth [1–5]. The recent explosion of research on nano- tion, according to which the shape is the inner convex structured materials depends heavily on the crystal growth hull bounded by planes (Wulff planes) [28–32]. When inor- theory and technology [6–18]. As a synergetic result of the ganic crystals are formed under equilibrium conditions, crystal structure and specific growth conditions, the crystal their crystal habit is determined by the relative order of sur- morphology comprehensively reflects the detailed growth face energies. Fast crystal growth will occur in the direction history and records the variation of growth conditions perpendicular to the face with the highest surface energy, [19–26]. The variously shaped crystals possess both aes- while, during real crystal growth process, branching thetic beauty and scientific attraction; natural gemstones, growth can be created by a diffusion effect. When a crystal for example, possess crystal morphologies and degrees of grows, ions or molecules near its surface are consumed by perfection that have yet to be duplicated by artificial pro- the growing crystal and a concentric diffusion field forms cess, while snowflakes decorate our world with beautiful around the crystal [33]. This makes the apexes of a polyhe- dral crystal, which protrude further into the region of higher concentration, grow faster than the central part of facets, thus forming branches. * Corresponding author. As a p-type semiconductor (direct bandgap 2.17 eV) E-mail address: [email protected] (D. Xue). with unique optical and magnetic properties, cuprous oxide 1359-6454/$30.00 Ó 2006 Acta Materialia Inc. Published by Elsevier Ltd. All rights reserved. doi:10.1016/j.actamat.2006.11.032 2398 J. Xu, D. Xue / Acta Materialia 55 (2007) 2397–2406 (Cu2O) is a promising material with potential applications was adjusted by adding NaOH solution when required. in solar energy conversion, magnetic storage devices, and Prior to the reaction, the mixture was sonicated in an catalysis [34–37]. Cuprous oxide possesses a cubic crystal ultrasonic water bath for 10 min. The bluish solution symmetry, and it has long been known that this solid com- was then transferred into a Teflon-lined stainless steel pound can be prepared into cubic, cuboctahedral and octa- autoclave, which was filled to 80% of its capacity hedral crystals in solution media [38,39]. Many recent (80 ml). The autoclave was heated to 80–150 °C for efforts have been devoted to the shape-controlled synthesis 6–24 h (detailed experimental conditions are shown in of Cu2O micro- and nanocrystals, such as electrodeposit- Table 1), and then allowed to cool to room temperature ion, thermal relaxation, sonochemical methods, vacuum naturally. The red products (as shown in the inset of evaporation and the liquid-phase reduction of a metal salt Fig. 6b) were collected and washed several times with dis- [40–47]. Herein, a novel reduction route has been demon- tilled water and absolute ethanol, respectively. The final strated by employing the reductant ethylenediaminetetra- products were dried at 50 °C (more than 5 h) for further acetic acid tetrasodium salt dihydrate (EDTA salt, characterizations. The phase and crystallographic struc- C10H12N2Na4O8 Æ 2H2O). ture of the as-prepared samples were determined by pow- The stability of the aqueous solution can be improved by EDTA chelating with Cu(II) cations (stability constant of Cu(II)–EDTA = 1018.8 at 20–25 °C), restraining precipi- Table 1 tation in the alkaline environment. Self-oxidation–reduc- A brief summary of representative experiments in this work tion reactions in the autoclave possibly take place under No. EDTA/Cu [Cu2+] pH Temperature Time Results hydrothermal conditions at elevated temperatures. In the molar ratio (°C) (h) current synthetic system, EDTA serves as both chelating 1 1 0.05 8 110 6 Fig. 7a1, a2 reagent and reductant, which is different from the previ- 2 1 0.05 8 110 12 Fig. 7a3, a4, Fig. 10i ously reported reductants for the synthesis of Cu2O (such 3 1 0.05 9 110 6 Fig. 7b1, b2 as glucose, formic acid, acetic acid, NaBH4 and DMF). 4 1 0.05 9 110 12 Fig. 7b3 Usually, organic acid can act not only as an acid, but also 5 1 0.05 9 110 24 Fig. 7b4 a reducing agent, a facility that has been widely investi- 6 1 0.05 9 120 6 Fig. 7c1, c2 gated in previous work [16,39]. At the same time, the deg- 7 1 0.05 9 120 12 Fig. 7c3 8 1 0.05 9 120 24 Fig. 7c4 radation (oxidation) of EDTA has been thoroughly 9 1 0.10 9 110 12 Fig. 7d1–d4 investigated in the fields of biochemistry and environmen- 10 1 0.10 9 120 24 Fig. 7e1, e2 tal chemistry, showing the final degradation products of 11 1 0.10 9 130 24 Fig. 7e3, e4 EDTA to be CO2 and H2O [48–51]. EDTA should be a reducing agent in the current synthetic system, since 12 1.5 0.05 8 110 12 Fig. 8a1, a2, c1, Fig. 10iii Cu2O microcrystals have been found at the end of the 13 1.5 0.05 8 110 24 Fig. 8a3, a4 experimental procedures. 14 1.5 0.05 9 110 12 Fig. 8b1, b2 Choi et al. [40–42] reported an electrochemical growth 15 1.5 0.05 9 110 24 Fig. 8b3, b4 16 1.5 0.10 10 110 24 Fig. 8c2–c4 route to obtain Cu2O microcrystals with a vast array of architectures, which indicates that Cu O is generally 17 1.5 0.10 11 110 12 Fig. 8d1, d2 2 18 1.5 0.10 11 110 24 Fig. 8d3, d4 selected as a model to investigate the shape evolutions in 19 1.5 0.10 12 110 12 Fig. 8e1, e2 the cubic crystal system. Usually, Cu2O architectures are 20 1.5 0.10 12 110 24 Fig. 8e3, e4, based on 6-, 8- or 12-pod branching growth patterns. In Fig. 10ii the current synthetic system, five branching growth pat- 21 1.5 0.10 13 120 12 Fig. 8f1, f2 terns have been proposed, which are the full range of 22 1.5 0.10 13 120 24 Fig. 8f3, f4 branching growth patterns for the cubic crystal system. 23 2 0.05 9 100 12 Fig. 9a1, a2 In particular, two kinds of high symmetric 24-pod branch- 24 2 0.05 10 100 12 Fig. 9a3, a4 ing growth patterns (i.e. 2 · 12-pod and 4 · 6-pod branch- 25 2 0.05 11 110 12 Fig. 9b1–b3, ing growth patterns) have not been reported previously in Fig. 10v any synthetic systems. 26 2 0.05 11 110 24 Fig. 9b4 27 2 0.10 12 110 12 Fig. 9c1, c2 28 2 0.10 12 110 24 Fig. 9c3, c4 2. Experimental 29 2 0.10 13 120 12 Fig. 9d1, d2 30 2 0.10 13 120 24 Fig. 9d3, d4 All reagents in the current experiments were of analy- 31 2 0.10 14 130 12 Fig. 9e1, e2 tical grade (purchased from Shanghai Chemical Industrial 32 2 0.10 14 130 24 Fig. 9e3, e4, Fig. 10iv Co.) and were used as-received without further purifica- tion. Solutions of Cu(II)–EDTA2À were prepared by dis- A series of experiments was also conducted in the present work. When the temperature was lower that 80 °C or pH < 8, the self-oxidation–reduction solving equimolar quantities of EDTA and Cu(NO3)2 in reaction could not take place. When the temperature was higher than deionized water or stoichiometric ratios of EDTA/Cu(II) 150 °C, some particles with irregular morphologies were observed. These (fixed as 1.5 and 2, respectively). The pH of the solutions data are therefore not included in the above table.
Details
-
File Typepdf
-
Upload Time-
-
Content LanguagesEnglish
-
Upload UserAnonymous/Not logged-in
-
File Pages10 Page
-
File Size-