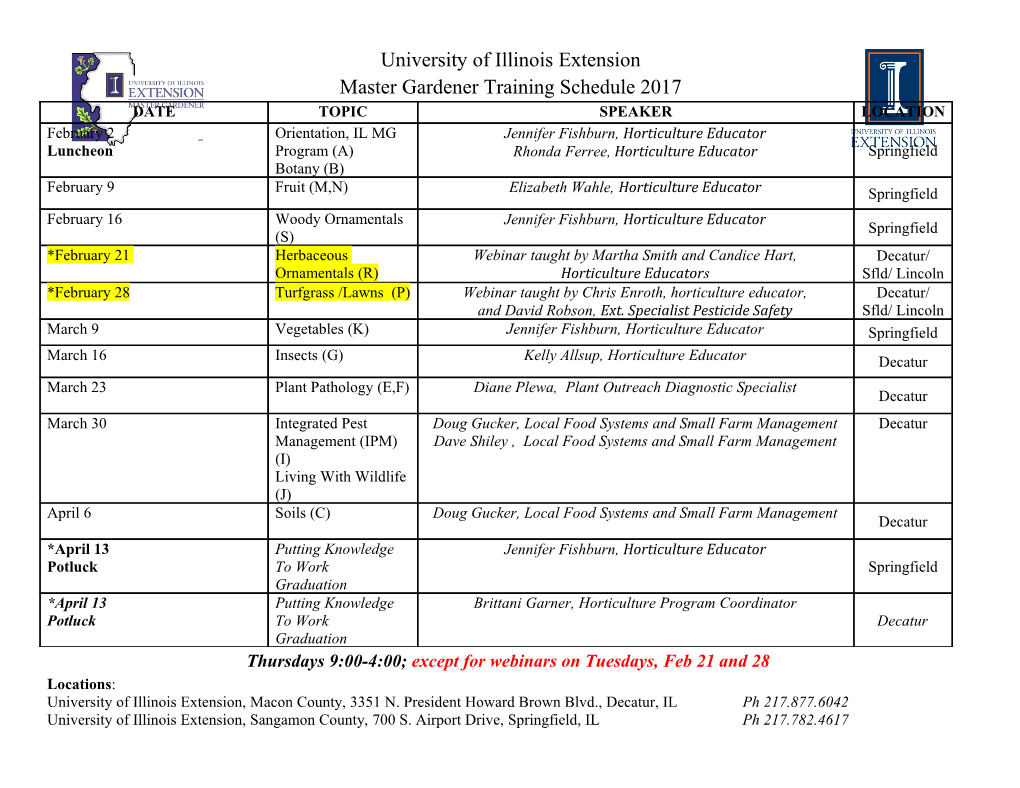
Available online at www.sciencedirect.com Conformational selection in trypsin-like proteases Nicola Pozzi, Austin D Vogt, David W Gohara and Enrico Di Cera For over four decades, two competing mechanisms of ligand recognition and regulation in trypsin-like proteases is recognition — conformational selection and induced-fit — relevant to many other biological systems. We therefore have dominated our interpretation of protein allostery. Defining start our discussion with the fundamental properties of the the mechanism broadens our understanding of the system and simplest allosteric models of ligand recognition — confor- impacts our ability to design effective drugs and new mational selection and induced-fit — and present an effec- therapeutics. Recent kinetics studies demonstrate that trypsin- tive experimental strategy to distinguish between them. like proteases exist in equilibrium between two forms: one fully accessible to substrate (E) and the other with the active site Conformational selection or induced-fit? occluded (E*). Analysis of the structural database confirms In its simplest incarnation, binding of ligand L to its existence of the E* and E forms and vouches for the allosteric biological target E can be cast in terms of the reaction nature of the trypsin fold. Allostery in terms of conformational (Scheme 1). selection establishes an important paradigm in the protease À1 À1 field and enables protein engineers to expand the repertoire of In Scheme 1 kon (M s ) is the second-order rate constant À1 proteases as therapeutics. for ligand binding and koff (s ) is the first-order rate of dissociation of the E:L complex into the parent species E Address and L. The strength of the interaction is quantified by the À1 Edward A. Doisy Department of Biochemistry and Molecular Biology, equilibrium association constant Ka (M ) defined as the Saint Louis University School of Medicine, St. Louis, MO 63104, United ratio k /k , or equivalently by the equilibrium dis- States on off sociation constant Kd (M) defined as the inverse of Ka, Corresponding author: Di Cera, Enrico ([email protected]) or koff/kon. Scheme 1 provides an important reference point for any discussion of ligand binding, but it offers little information about the mechanism of recognition. What Current Opinion in Structural Biology 2012, 22:421–431 happens when the ligand binds to its target? Is the inter- This review comes from a themed issue on Engineering and action a rigid body collision between preconfigured species design or does it involve conformational changes that optimize Edited by Jane Clarke and William Schief binding? In the former case, Scheme 1 adequately For a complete overview see the Issue and the Editorial describes the kinetics of interaction and the system approaches equilibrium according to an observed rate Available online 3rd June 2012 constant, kobs that increases linearly with [L]. In the latter, 0959-440X/$ – see front matter, # 2012 Elsevier Ltd. All rights the dependence of kobs on [L] is not linear and kobs depends reserved. on the nature of conformational changes involved [4 ]. http://dx.doi.org/10.1016/j.sbi.2012.05.006 Two limiting cases become of interest as the simplest interpretations of allostery linking binding to confor- mational transitions [1 ]. In the first case (Scheme 2), Introduction the target exists in distinct conformations in equilibrium Nearly all biological activities of a cell depend on the and the ligand selects the one with optimal fit. specific encounter between a ligand and a host target. Understanding the molecular mechanism of how ligands The species E* is added to reflect a pre-existing equi- recognize their biological targets and how interactions are librium between two forms, E* and E, of which only E regulated remains a central issue to biology, biochemistry, can interact with the ligand L. This is the simplest form of and biophysics [1 ]. The importance extends beyond the the celebrated Monod–Wyman–Changeux model of allo- basic sciences and affects our ability to design effective steric transitions [1 ]. In the second case (Scheme 3), the drugs and new therapeutics. Knowledge recently conformation of the target changes after ligand binds to emerged from rapid kinetics and structural biology stu- provide an optimal fit. dies on a large class of enzymes, the trypsin-like proteases [2 ], reveals the unique role that allostery plays in these Scheme 3 is the simplest form of the alternative Kosh- proteins as the basic mechanism of function and regula- land–Nemethy–Filmer model of allosteric transitions tion. Protein engineering strategies exploiting the allo- [1 ]. The two mechanisms above can be combined in steric nature of the trypsin fold show promising new a more realistic version of ligand binding where a pre- translational opportunities that expand the existing land- existing equilibrium is followed by ligand-induced con- scape of proteases as therapeutics [3 ]. A critical account formational changes. However, it is quite instructive to of how allostery emerged as the key mechanism of ligand note the properties of the simple Schemes 2 and 3 in the www.sciencedirect.com Current Opinion in Structural Biology 2012, 22:421–431 422 Engineering and design Scheme 1 Scheme 3 k koff k-r off ∗ ∗ E E:L E E :L E:L kon[L] kon [L] kr Scheme 2 to the conformational changes, that are therefore rate- limiting [4 ]. This is the scenario where the contribution k-r koff ∗ of any conformational change to the kinetics of approach to E E E:L equilibrium can be best evaluated. The case where con- kr kon [L] formational changes are rapid compared to binding and dissociation is of little interest because it turns Schemes 2 kinetics of approach to equilibrium, where invaluable and 3 into Scheme 1. The kinetic properties of Schemes 2 information can be gathered about the nature of confor- and 3 in the general case are of great interest, but beyond mational transitions involved. the scopes of this review and will be discussed elsewhere. Under the assumption of rate-limiting conformational A convenient and widely used assumption is that binding changes, the dependence of kobs on [L] becomes diagnostic and dissociation steps in Schemes 2 and 3 are fast compared of the mechanism involved (Figure 1). In the case of Figure 1 k [L] ∗ on E E∗ E∗L koff k k -r r k-r kr kon [L] E EL EL koff 60 60 55 k-r + kr 55 k-r + kr 50 50 45 45 40 K k = k + k d 40 ) obs r –r ) -I 35 -I 35 Kd + [L] (s 30 (s 30 [L] obs 25 obs 25 k = k + k k k obs –r r K + [L] 20 20 d 15 15 10 10 5 Kd Kd kr 5 k-r 0 0 0 1 2 3 4 5 6 7 8 910 0 1 2 3 4 5 6 7 8 910 [L] (μM) [L] (μM) Conformational Selection Induced-Fit Current Opinion in Structural Biology Conformational selection and induced-fit. Under the assumption of rate-limiting conformational changes, the dependence of kobs on the ligand concentration is diagnostic of the mechanism of binding, that is, Scheme 2 or Scheme 3. Conformational selection (top left) postulates a pre-existing equilibrium between the E* and E forms, of which only E binds the ligand. The rate of approach to equilibrium, kobs, is an inverse hyperbolic function of the ligand concentration (bottom left, yellow inset) from which the values of kr and kÀr for the E*–E interconversion can be derived directly, along with the value of Kd for ligand binding. In this mechanism, the value of kobs decreases with increasing ligand concentration. The induced-fit model (top right) postulates a conformational transition between E*:L and E:L that optimizes binding. The rate of approach to equilibrium, kobs, is an hyperbolic function of the ligand concentration (bottom right, yellow inset) from which the values of kr and kÀr for the E*:L–E:L interconversion can be derived directly, along with the value of Kd for ligand binding. In this mechanism, the value of kobs increases with increasing ligand concentration. Current Opinion in Structural Biology 2012, 22:421–431 www.sciencedirect.com Conformational selection in trypsin-like proteases Pozzi et al. 423 Scheme 3, the kobs increases hyperbolically with [L], a Trypsin-like proteases are allosteric enzymes situation analogous yet not identical to that seen in the obeying conformational selection simple Scheme 1. In the case of Scheme 2, the kobs decreases Trypsin-like proteases utilize a catalytic triad for activity, hyperbolically with [L], a situation that unambiguously composed of the highly conserved residues H57, D102, signals the presence of pre-equilibrium between two con- and S195 (chymotrypsinogen numbering). Catalysis is formations and rules out both Schemes 1 and 3. Hence, assisted by several structural determinants surrounding conformational selection (Scheme 2) has unique kinetic the active site [14]. Residue 189 at the bottom of the signatures that, once detected experimentally, leave no primary specificity pocket engages the substrate residue room for alternative explanations based on Scheme 3 or at the site of cleavage. The oxyanion hole defined by the Scheme 1. This is exactly what emerged recently in the backbone N atoms of S195 and G193 stabilizes the analysis of the kinetic mechanism of ligand binding to developing partial charge on the tetrahedral intermediate thrombin (Figure 2) [5 ,6 ], meizothrombin desF1 [7], during the catalytic cycle. Residues in the 215–217 seg- trypsin [8], clotting factor Xa, and activated protein C [9]. ment flanking the active site provide additional recog- Scheme 2 is obeyed in all cases and Schemes 1 and 3 are nition sites for substrate residues immediately upstream automatically ruled out. The ‘alternative’ proposal by of the peptide bond to be cleaved.
Details
-
File Typepdf
-
Upload Time-
-
Content LanguagesEnglish
-
Upload UserAnonymous/Not logged-in
-
File Pages11 Page
-
File Size-