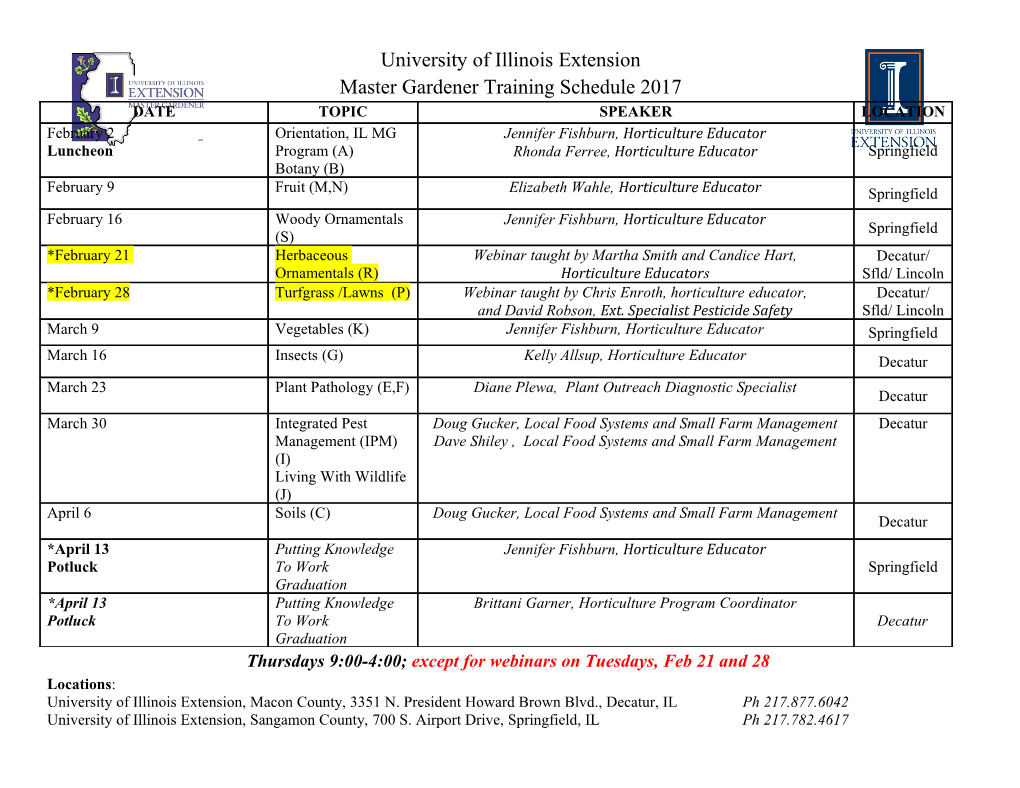
The Effects of Noise on Aquatic Life Den Haag, NL, 2019 Book of Abstracts 1 The Effects of Noise on Aquatic Life Den Haag, NL, 2019 INTERNATIONAL STANDARDIZATION IN UNDERWATER BIOACOUSTICS Michael A. Ainslie*1, Stephen P. Robinson2, Michele B. Halvorsen3, Christ A. F. de Jong4 1 JASCO Applied Sciences, Eschborn, Germany, email: [email protected] 2 NPL, Teddington, United Kingdom, email: [email protected]. 3 CSA Ocean Sciences Inc., Stuart (Florida), USA, email: [email protected] 4 TNO, The Hague, The Netherlands, email: [email protected] * presenting author Standards are helpful in all walks of life, from buying a plug that fits that socket at home to synchronizing a docking operation at the International Space Station at 15:58:22 UTC. Standardization in air acoustics began as an initiative of the American Standards Association (now ANSI) in the 1930s, and the International Organization for Standardization (ISO) established its technical committee Acoustics (TC 43) in 1947. Seventy years later we can buy a standardized sound level meter knowing that it applies an appropriate auditory filter, tuned to the perception of human hearing. In underwater acoustics the process of international standardization is in its infancy. For example, as of 2019 there exists no standard hearing filter for a single species of aquatic animal, no international standard for measuring sound pressure or particle motion, or hearing thresholds, source levels or scattering strengths of aquatic biota. ISO TC 43’s first step towards standardization in underwater acoustics was made in 2011 when it established the sub-committee Underwater Acoustics (TC 43/SC 3). Since then, reference values (ISO 1683:2015) and terminology (ISO 18405:2017) in underwater acoustics have been standardized, SC 3 has published two measurement standards (ISO 17208-1:2016; ISO 18406:2017), and the International Electrotechnical Commission (IEC) has updated its hydrophone specification (IEC 60500:2017). As part of a major revision of the International System of Quantities, ISO 80000-8 ‘Quantities and units -- Part 8: Acoustics’ is being updated to include underwater acoustics, with the revised standard scheduled for publication in 2019. The Working Group on Standardization for the International Quiet Ocean Experiment has published an inventory of existing standards for observations of sound in the ocean (https://iqoe.org/groups/standardization). Example applications include the standardization project of the E&P Sound and Marine Life Joint Industry Programme, and several underwater sound monitoring projects, including ADEON (https://adeon.unh.edu/), JOMOPANS (https://northsearegion.eu/jomopans/), and QUIETMED (http://www.quietmed-project.eu/). The development and application of international standards in underwater acoustics since 2015, and new international standards currently under development, are described, with special attention to their application to the protection of aquatic life. Areas where international standards are lacking are described. Such standards are needed to facilitate inter-laboratory, inter-disciplinary and international compatibility, and to avoid costly mistakes. Without them scientific progress is hindered by the lack of inter-project compatibility, resulting in effort wasted by repeatedly re-inventing the wheel. IEC (2017). IEC 60500:2017 “Underwater acoustics – Hydrophones – Properties of hydrophones in the frequency range 1 Hz to 500 kHz,” International Electrotechnical Commission (Geneva). ISO (2016). ISO 17208-1:2016 “Underwater acoustics -- Quantities and procedures for description and measurement of underwater sound from ships -- Part 1: Requirements for precision measurements in deep water used for comparison purposes,” International Organization for Standardization (Geneva). ISO (2017a). ISO 18405:2017 “Underwater acoustics -- Terminology,” International Organization for Standardization (Geneva). ISO (2017b). ISO 18406:2017 “Underwater acoustics -- Measurement of radiated underwater sound from percussive pile driving,” International Organization for Standardization (Geneva). 2 The Effects of Noise on Aquatic Life Den Haag, NL, 2019 APPLICATION OF DAMPED CYLINDRICAL SPREADING TO IMPACT PILE DRIVING RISK ASSESSMENT Michael A. Ainslie*1, Michele B. Halvorsen2, Roel A. Müller3, Tristan Lippert4 1 JASCO Applied Sciences, Eschborn, Germany, email: [email protected] 2 CSA Ocean Sciences Inc., Stuart (Florida), USA, email: [email protected] 3 TNO, The Hague, The Netherlands, email: [email protected] 4 Hamburg University of Technology, Hamburg, Germany, email: [email protected] Environmental risk assessment for impact pile driving requires characterization of the radiated sound field. A widely used method to estimate the field at range is to measure the sound exposure level (SEL) at (say) �1 and extrapolate from �1 to � using an empirical formula for transmission loss (TL) of the form 15log(�/�1) dB, or similar. A recently developed alternative to this “15logR” approach is a theoretical model called damped cylindrical spreading (DCS) (Lippert et al., 2018). DCS describes sound propagation of the Mach cone generated by the interaction of the hammer with the pile (Reinhall and Dahl, 2011) and further associates the TL with the Mach cone, which naturally follows cylindrical spreading combined with exponential decay caused by multiple seabed reflections (Zampolli et al., 2013). For impact assessment it is conventional to also use criteria involving peak sound pressure level (Lpk) and root- mean-square sound pressure level (Lrms). Lpk and Lrms can be estimated from SEL using empirical correlations based on (Lippert et al., 2015). A regression analysis was carried out on measurements for four wind farm construction sites to produce correlations representative of the southern North Sea, thus facilitating predictions using the DCS approach of SEL, Lpk and Lrms. The traditional 15logR and alternative DCS model methods were compared with measurements made during the construction of Borkum Riffgrund I. In this validation test, the 15logR approach overestimated the initial slope of the TL curve, and consequently underestimated SEL for distances between 30 m and 3 km, whereas the DCS prediction showed a better agreement with the measurements. This improved accuracy is explained by the use by DCS of basic physical principles, leading to cylindrical spreading of sound trapped by the shallow water waveguide, from which it follows that if is chosen close to the pile the 15logR approach necessarily underestimates the impact. We illustrate this point by applying both methods to estimate impact on fish and harbour porpoise of the construction of a generic pile. When estimating the impact area associated with recoverable injury in fish, the 15logR approach predicted an impact distance of 0.19 km (area A = 0.11 km2), while DCS predicted 0.50 km (A = 0.79 km2). The 15logR approach therefore underestimated the impact area by a factor 7. For a similar calculation for the harbour porpoise, the 15logR approach underestimated the impact area for TTS in the harbour porpoise by a factor 4. Lippert, T., Ainslie, M. A., & von Estorff, O. (2018). "Pile driving acoustics made simple: Damped cylindrical spreading model," J. Acoust. Soc. Am. 143, 310-317. Lippert, T., Galindo-Romero, M., Gavrilov, A. N., & von Estorff, O. (2015). "Empirical estimation of peak pressure level from sound exposure level. Part II: Offshore impact pile driving noise," J. Acoust. Soc. Am. 138, EL287-EL292. Reinhall, P. G., & Dahl, P. H. (2011). "Underwater Mach wave radiation from impact pile driving: Theory and observation," J. Acoust. Soc. Am. 130, 1209-1216. Zampolli, M., Nijhof, M. J., de Jong, C. A., Ainslie, M. A., Jansen, E. H., & Quesson, B. A. (2013). "Validation of finite element computations for the quantitative prediction of underwater noise from impact pile driving," J. Acoust. Soc. Am. 133, 72-81. 3 The Effects of Noise on Aquatic Life Den Haag, NL, 2019 CETACEAN RESPONSE TO BROADBAND EXPLOSIVE NOISE IN KAUAI Gabriela Alongi*1, E. Elizabeth Henderson2, Stephen W. Martin3, Cameron Martin4, Tyler Helble5, Brian Matsuyama6 1 National Marine Mammal Foundation (NMMF), San Diego, CA, email: [email protected] 2 Naval Information Warfare Center (NIWC) Pacific, San Diego, CA, email: [email protected] 3 NMMF, San Diego, CA, email: [email protected] 4 NMMF, San Diego, CA, email: [email protected] 5 NIWC Pacific, San Diego, CA, email: [email protected] 6 NMMF, San Diego, CA, email: [email protected] Passive acoustic monitoring of marine mammals occurs regularly on the Pacific Missile Range Facility (PMRF) in Kauai, Hawaii. Disturbance analyses have been performed for some species in response to Navy training events, such as the biannual Submarine Command Course event and other periods of active sonar (e.g. Martin et al. 2015, Henderson et al. 2016, Manzano-Roth et al. 2016). Explosions have been opportunistically recorded in some datasets and contain enough acoustic energy to propagate large distances to be heard on multiple hydrophones across the ~1,100 km2 area of the PMRF instrumented range. Automated tracks were generated for minke whales (Balaenoptera acutorostrata), low-frequency baleen whales (including fin whales (Balaenoptera physalus) and Bryde’s whales (Balaenoptera edeni)), and humpback whales (Megaptera novaeangliae). These tracks form the basis to assess presence and, in
Details
-
File Typepdf
-
Upload Time-
-
Content LanguagesEnglish
-
Upload UserAnonymous/Not logged-in
-
File Pages223 Page
-
File Size-