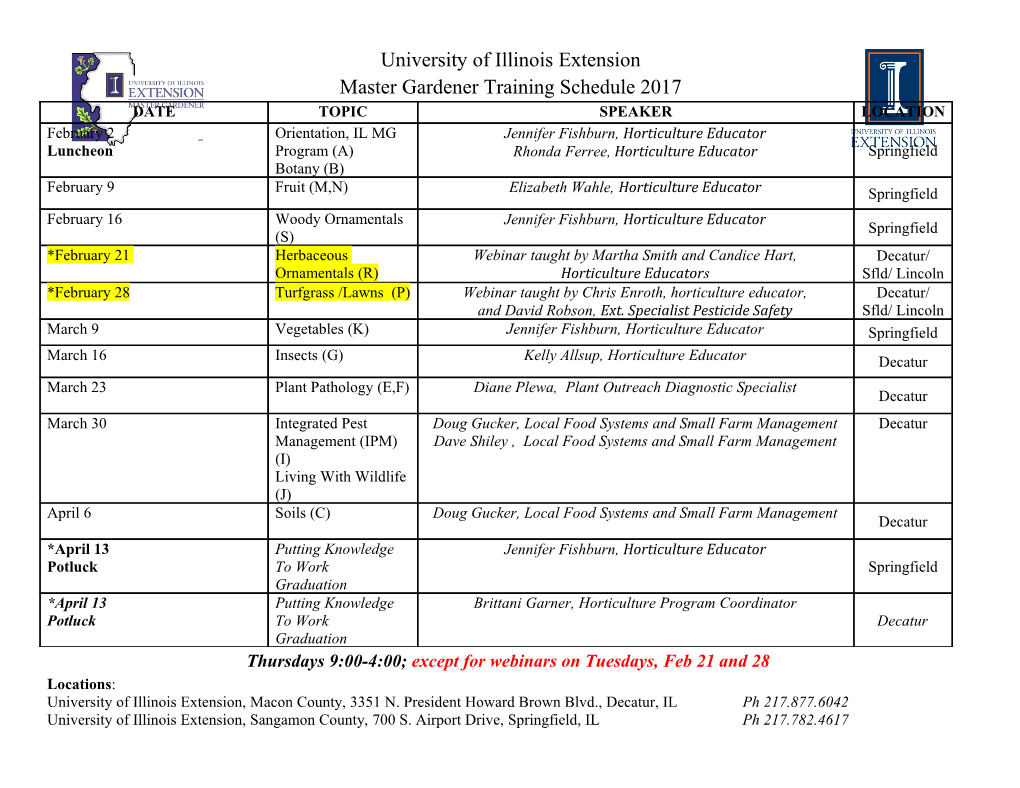
Multimodal gradients across mouse cortex Ben D. Fulchera,1, John D. Murrayb, Valerio Zerbic, and Xiao-Jing Wangd,e,1 aSchool of Physics, Sydney University, Sydney, NSW 2006, Australia; bDepartment of Psychiatry, Yale University School of Medicine, New Haven, CT 06511; cNeural Control of Movement Laboratory, Department of Health Sciences and Technology, Eidgenossische¨ Technische Hochschule Zurich,¨ 8057 Zurich,¨ Switzerland; dCenter for Neural Science, New York University, New York, NY 10003; and eShanghai Research Center for Brain Science and Brain-Inspired Intelligence, Shanghai 201210, China Edited by Robert Desimone, Massachusetts Institute of Technology, Cambridge, MA, and approved January 22, 2019 (received for review August 16, 2018) The primate cerebral cortex displays a hierarchy that extends from The ratio of T1-weighted to T2-weighted (T1w:T2w) images primary sensorimotor to association areas, supporting increas- is a noninvasive MRI measurement that has been measured in ingly integrated function underpinned by a gradient of hetero- mice, macaques, and humans, providing a common spatial refer- geneity in the brain’s microcircuits. The extent to which these ence map for linking large-scale cortical gradients across species. hierarchical gradients are unique to primate or may reflect a Commonly interpreted as a marker of intracortical myelin con- conserved mammalian principle of brain organization remains tent (45), T1w:T2w is also sensitive to a wide range of other unknown. Here we report the topographic similarity of large-scale microstructural properties (46). In macaque cortex, T1w:T2w gradients in cytoarchitecture, gene expression, interneuron cell is strongly correlated to the established structural hierarchy of densities, and long-range axonal connectivity, which vary from feedforward–feedback interareal laminar projections (47), and primary sensory to prefrontal areas of mouse cortex, highlighting in human cortex it follows dominant gene transcriptional gradi- an underappreciated spatial dimension of mouse cortical special- ents, positioning it as a strong candidate marker of hierarchical ization. Using the T1-weighted:T2-weighted (T1w:T2w) magnetic specialization (25). Here we show that gradients of diverse prop- resonance imaging map as a common spatial reference for com- erties of mouse cortex exhibit a common spatial patterning along parison across species, we report interspecies agreement in a a candidate functional hierarchy. We use T1w:T2w as a common range of large-scale cortical gradients, including a significant spatial reference to demonstrate a correspondence of gradients correspondence between gene transcriptional maps in mouse cor- of cytoarchitecture between mice, macaques, and humans and tex with their human orthologs in human cortex, as well as with transcriptional maps of ortholog genes between mice and notable interspecies differences. Our results support the view of humans. Our results reveal an interspecies conservation of cor- NEUROSCIENCE systematic structural variation across cortical areas as a core orga- tical gradients that may shape the functional specialization of nizational principle that may underlie hierarchical specialization mammalian cortical circuits, consistent with systematic structural in mammalian brains. variation across cortical areas as a core organizing principle (48). cortical hierarchy j gene expression j cortical gradients j Results interspecies comparison We analyzed the spatial maps of diverse cortical properties across 40 areas of the Allen Reference Atlas (ARA) (49), shown cross the brain, cortical microcircuits vary in their cytoarchi- in Fig. 1 A and B. The similarity between two spatial maps was Atecture (1–3); myeloarchitecture (3–5); dendritic and synap- quantified as the Spearman rank correlation coefficient, ρ, across tic structure (6–13); and the size, density, and laminar distribution as many cortical areas as could be matched between a given pair of distinct cell types (1, 3, 14–16). Many of these microstructural of modalities (40 unless otherwise specified). Corrected P val- properties vary across the cortex continuously as spatial gradients ues, Pcorr, are calculated using the method of Benjamini and (1–3, 17–20) that shape the specialized functional capabilities of Hochberg (50). different cortical areas (7), through variations in plasticity (5), inhibitory control (21), and electrophysiological properties (6, Significance 10). Prominent gradients follow a hierarchy of increasing func- tional integration, from primary sensory to transmodal associ- The brain is organized into processing streams, along which ation areas in primate cortex, pointing to their role in shaping incoming sensory information is processed at increasingly functional specialization along the cortical hierarchy (22–25). abstract and integrative levels. This specialization of function The mouse cortex is relatively uniform compared with the highly is thought to be underpinned by corresponding changes in the differentiated primate cortex (10, 26–28), but recent evidence has brain’s local circuitry. Here we combine a wide range of mea- nevertheless pointed to a global hierarchy of mouse cortical areas surements across the mouse brain, including the expression of (29, 30). It remains unknown whether the hierarchical gradients genes, cell densities, and axonal connectivity, to show that the of primate cortex exist and play a similar role in functional spe- properties of local circuits of the mouse brain vary along puta- cialization in the mouse and may therefore represent a conserved tive processing streams in a similar way to the primate brain. property of mammalian brain organization. This similarity across species points to common mechanisms The mouse is an ideal model to investigate gradients of cortical through which mammalian brains may process information. microstructure, with experimental datasets from diverse modal- ities available in standardized anatomical reference spaces (31). Author contributions: B.D.F., J.D.M., and X.-J.W. designed research; B.D.F. and V.Z. per- Cortical maps of a wide range of properties, many of which are formed research; J.D.M. and X.-J.W. contributed new reagents/analytic tools; B.D.F. and unavailable in humans, have been measured in mice, including (i) V.Z. analyzed data; and B.D.F., J.D.M., and X.-J.W. wrote the paper.y gene expression with approximate genome-wide coverage (32), The authors declare no conflict of interest.y (ii) interneuron cell densities (29), (iii) tract-traced axonal con- This article is a PNAS Direct Submission.y nectivity (30, 33–36), (iv) cytoarchitecture (37), (v) cell/neuron Published under the PNAS license.y density (38–40), and (vi) resting-state function magnetic reso- 1 To whom correspondence may be addressed. Email: [email protected] or nance imaging (fMRI) (41–43). Existing work has demonstrated [email protected] an association between pairs of these measurements (42–44), but This article contains supporting information online at www.pnas.org/lookup/suppl/doi:10. these data have not previously been characterized together from 1073/pnas.1814144116/-/DCSupplemental.y the viewpoint of macroscopic gradients. Published online February 19, 2019. www.pnas.org/cgi/doi/10.1073/pnas.1814144116 PNAS j March 5, 2019 j vol. 116 j no. 10 j 4689–4695 Downloaded by guest on October 1, 2021 T1w:T2w A 1.18 RSPd B RSPagl VISamVISpm SSp-tr 1.1 SSp-ll PTLp VISp somatomotor 0.60 SSp-ul 1 medial SSp-bf VISal SSp-un VISl temporal ACAd visual AUDpo VISpl anterolateral PL MOs AUDd 0.9 MOp SSp-n FRP AUDp prefrontal SSp-m T1w:T2w 0.8 ORB SSs AUDv TEa 0.7 ECT Superior AId GU VISC PERI 0.6 Right Posterior PL AIv GU AIv AId ApI ILA TEa AIp SSs VISl Anterior ECT FRP MOs MOp VISp VISC Left PERI ORBl VISpl PTLp VISal RSPv ACAv AUDv RSPd ACAd AUDp SSp-ll AUDd SSp-n SSp-tr ORBvl ORBm VISpm SSp-ul VISam Inferior SSp-m AUDpo SSp-un RSPagl SSp-bfd Cortical area 10-3 0.7 7 40 ACAd AUDp SSp-bfd SSp-m SSp-n AIv C D E AId F TEa 4 MOp SSp-ul SSp-bf 35 MOs d 6 ACAv VISC SSp-m 0.6 VISpl VISp SSp-ll VISp SSs ORBvl SSp-un ORBl 30 SSp-tr VISal AUDpo VISam SSs RSPd 5 AUDv SSp-tr SSp-ll AUDd 0.5 AUDp AUDd ORBl VISpm 3 ACAv ACAd MOs PTLp 25 PL VISC SSp-ul FRP FRP SSp-n PTLp AId VISC 4 PL RSPagl VISpl MOs VISal FRP VISam ORBvl RSPv AId VISal 0.4 RSPagl ORBvl 20 VISl VISam GU AUDpo ILA ORBm VISpm 3 VISpl ORBl RSPd SSp-tr GU AIv AUDv VISpm 2 AIv AUDp 15 MOp TEa 0.3 ORBm TEa GU MOp VISl ORBm TEa SSp-bfd 2 VISC MOs VISal RSPv SSs Cytoarchitecture type AIp VISp 10 ORBvl AId ILA PERI RSPaglAUDv VISam VISpl SSp-m PL SSp-n PTLp Inferred hierarchical level ACAv AIp ORBl RSPv 0.2 ECT RSPd AUDd RSPd FRP ILA ACAv PERI VISpm AUDd AIp SSp-ul 1 AIp 1 SSp-ul SSs 5 PV:(PV+SST) cell density (L2/3) VISl SSp-m SSp-ll ORBm AIv ACAd ACAd SSp-bfd SSp-n ILA PLECT RSPagl Afferent axonal connection density RSPv SSp-tr VISp PERI 0.1 0 SSp-ll 0 AUDp 0.7 0.8 0.9 1.0 1.1 0.7 0.8 0.9 1.0 1.1 0.7 0.8 0.9 1.0 1.1 0.7 0.8 0.9 1.0 1.1 T1w:T2w T1w:T2w T1w:T2w T1w:T2w Fig. 1. The spatial map of the MRI measurement, T1w:T2w, is correlated with spatial maps of other structural properties. (A) Variation in T1w:T2w across mouse cortical areas (visualization through medial sections is in SI Appendix, Fig. S3). (B) T1w:T2w broadly decreases across families of connectivity-based groupings of cortical areas (30), from somatomotor areas to anterolateral and prefrontal areas. Groups are ordered by decreasing T1w:T2w, as are areas within each group. (C–F) Scatter plots are shown for T1w:T2w (horizontal axis) vs.
Details
-
File Typepdf
-
Upload Time-
-
Content LanguagesEnglish
-
Upload UserAnonymous/Not logged-in
-
File Pages7 Page
-
File Size-