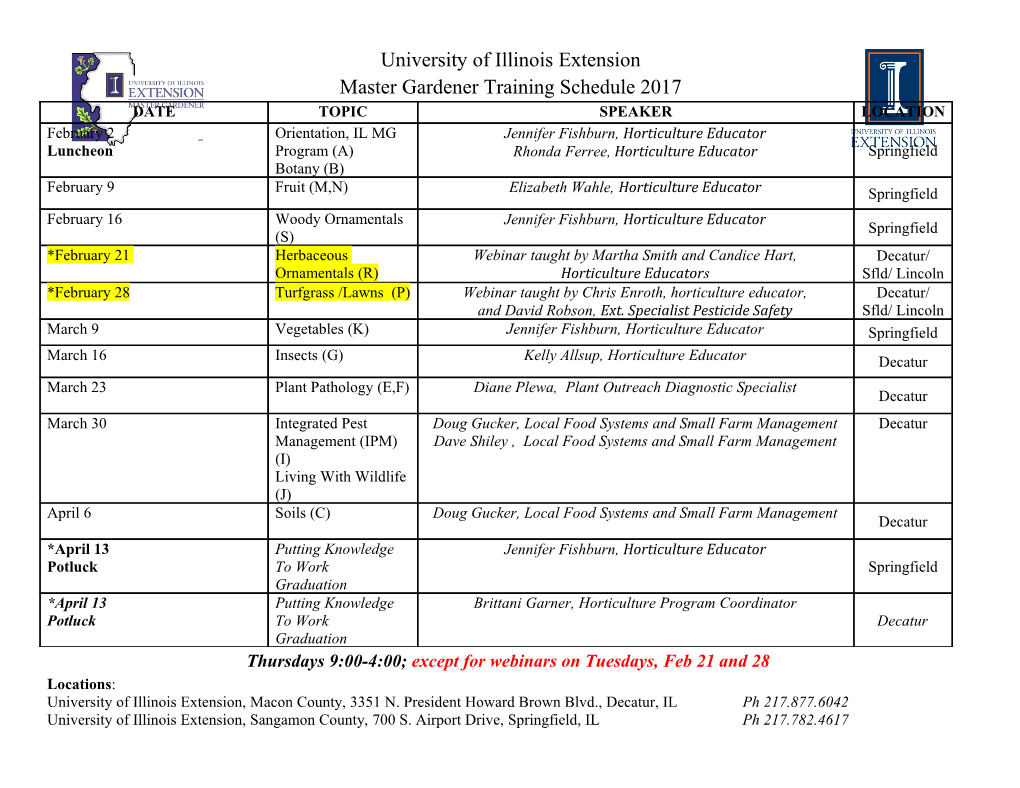
1.4 Solar cell losses and design In this final introduction video on photovoltaic energy conversion, we will discuss the various parts of a solar cell and the losses that occur in a solar cell. The losses in solar cells will provide an important framework to put everything we learn over the course of the next couple of weeks in context. The learning objective for this video are to understand the main function of the various parts of a solar cell. We will discuss the main losses that occur in solar cells and we will come to understand how these losses lead to the design rules for solar cells. Shown here is a standard silicon wafer based solar cell. These are the most common type of solar cells, accounting for about 93 % of the total production in 2015. We will base this solar cell on a p-type silicon absorber even though some silicon cells can be made with an n-type absorber layer. The purpose of this absorber layer, as its name implies, is to absorb light. Through this absorption, minority and majority charge charge carriers are formed. In the case of a p-type absorber, electrons are the minority carriers and holes are the majority carriers. Next is the emitter layer. The emitter layer is crucial for charge carrier separation and collection. The emitter layer functions as an selective membrane, that allows minority charge carriers, in this case electrons, to move through, but resists the movement of majority carriers, in this case holes. Without the emitter layer, generated charge carriers would simply move around in the absorber layer until they recombine. Similarly, at the back of the solar cell we have the back surface field, or BSF. This acts as a semipermeable membrane for majority charge carriers, in this case holes. With the BSF and the emitter we can separate electrons and holes to either side of the solar cell. In order to collect these carriers and send them to an external circuit, metallic contacts, or electrodes, are needed at both sides of the solar cell. When the solar cell is connected to an external circuit, the excited electrons will flow through the circuit to recombine with holes at the other electrode. Only a small fraction of the front surface is covered by electrodes. Charge carriers therefore have to move laterally to the front electrode. In a high quality c-Si solar cell, this lateral charge carrier movement takes place in the emitter layer. For many PV technologies however, the conductive properties of the emitter layer are too poor for good lateral transport. For these technologies a transparent conductive oxide layer is introduced, that is transparent to the incident light, but has excellent conductive properties, to facilitate the lateral movement of charge carriers to the front electrode. Finally, an anti-reflection coating is placed at the front of the cell, to reduce the front surface reflection, and maximize the light transmission into the cell. For the last seventy years, the performance of crystalline silicon solar cells has steadily increased. The efficiency of solar cells, with an area smaller than 5 square centimeters, is plotted here over a period of 80 years. The red data points represent crystalline silicon solar cells with an n-type absorber, while the blue data points are cells with a p-type absorber layer. Over the last 15 years the performance of solar cells with a larger area, up to 15 square centimeter, have also strongly increased. The performance of the crystalline silicon solar cells is still steadily increasing, up to the theoretical limit for crystalline silicon solar cells, of 29.4 %. We will learn more about the theoretical limits of solar cell performance in the final week of this course. The closer we get to this limit, the harder it will be to further improve the performance of the cells. The current record efficiency, as of december 2016, of single junction crystalline silicon solar cells is set at 26.3%, by the Kaneka corporation. This still means however that 73.7% of the power incident on a solar cell is lost. Let’s take a look at these losses. Each of the loss mechanisms will be extensively discussed in the following sections on optics and semiconductor physics. The losses that occur in solar cells can be divided into optical losses and electrical losses. By far the largest losses occur due to spectral mismatch. Spectral mismatch considers the notion that, of the incident solar spectrum, some of the photons with a low energy level can not be absorbed by a single junction solar cell. Of other photons, with a high energy level, only a fraction of the energy of a photon can be used. Additional losses further limit the optical performance of a solar cell. Among these are the reflection of light from the front surface of a solar cell, and the transmission of light from the cell that is not absorbed. Additionally, we will learn about parasitic absorption and shading losses. The additional optical losses and spectral mismatch losses together make up the optical losses part. In the electrical losses section, we consider the recombination losses and other losses. As we have discussed, recombination losses occur when an electron, after excitation, falls back to its initial energy state. If this happens, that absorbed energy is essentially lost and cannot be converted into electrical energy. We will discuss three special cases of recombination in the section on semiconductor physics. Finally, there are the other electrical losses. These involve the resistive losses and shunt losses we discussed in the video on the equivalent circuit of the solar cell. So this is what the full overview of losses in any type of solar cell looks like. We can translate these loss mechanisms into the three major solar cell design rules. The losses due the spectral mismatch are the subject of the spectral utilization. The additional optical losses are the main concern of the design rule known as light management. The electrical losses are considered in the bandgap utilization design rules. Designing any type of a solar cell involves a delicate interplay between these three pillars. In summary, we discussed the basic function of each of the layers in a standard crystalline silicon solar cell. We looked at an overview of the loss mechanisms that occur in any type of solar cell and we derived from these the solar cell design rules. You have now come to the end of the last video of the introductory week. In the rest of this course we will dive deeply into the physical and optical principles behind these loss mechanisms and learn methods to tackle them as a photovoltaic engineer. .
Details
-
File Typepdf
-
Upload Time-
-
Content LanguagesEnglish
-
Upload UserAnonymous/Not logged-in
-
File Pages4 Page
-
File Size-