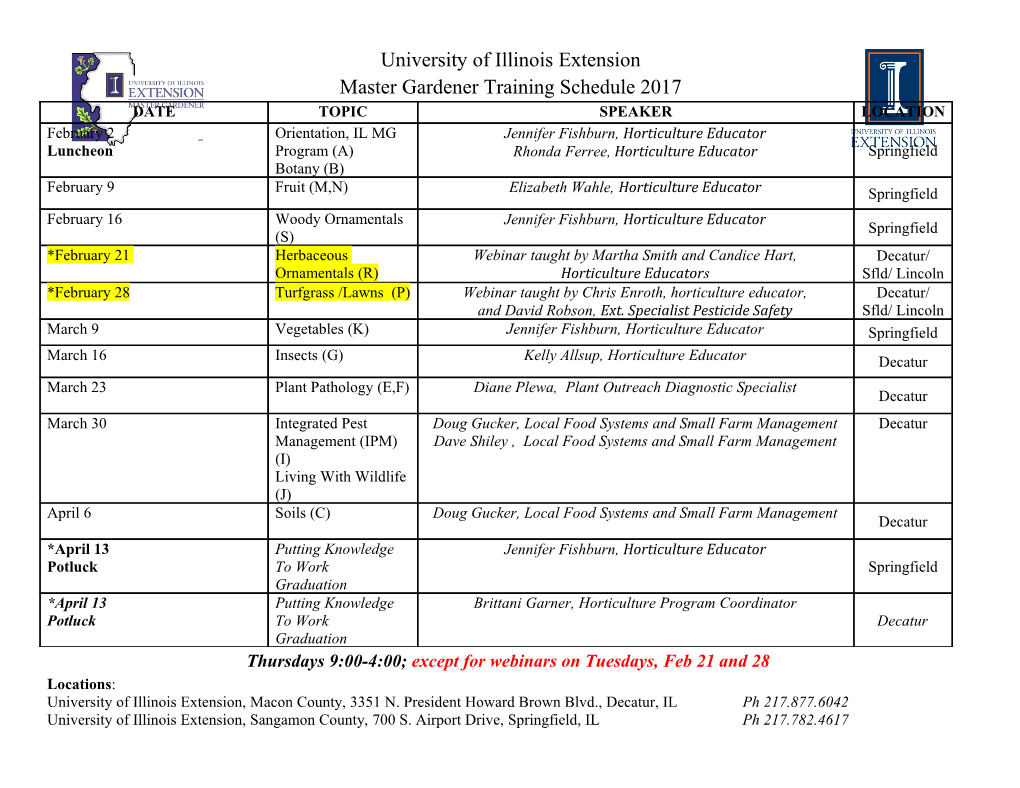
The effector mechanism of siRNA spherical nucleic acids Gokay Yamankurta,b,c,d, Robert J. Stawickib,c, Diana M. Posadasd, Joseph Q. Nguyend, Richard W. Carthewd,e,1, and Chad A. Mirkinb,c,1 aInterdisciplinary Biological Sciences Graduate Program, Northwestern University, Evanston, IL 60208; bInternational Institute for Nanotechnology, Northwestern University, Evanston, IL 60208; cDepartment of Chemistry, Northwestern University, Evanston, IL 60208; dDepartment of Molecular Biosciences, Northwestern University, Evanston, IL 60208; and eNSF-Simons Center for Quantitative Biology, Northwestern University, Evanston, IL 60208 Contributed by Chad A. Mirkin, November 26, 2019 (sent for review September 13, 2019; reviewed by Daniel G. Anderson and Erik J. Sontheimer) Spherical nucleic acids (SNAs) are nanostructures formed by chem- SNAs (13–16). Specifically, it is not clear whether dissociation of ically conjugating short linear strands of oligonucleotides to a RNA from the SNA is required for gene silencing and whether the nanoparticle template. When made with modified small interfering SNA acts through the canonical RNAi pathway. RNA (siRNA) duplexes, SNAs act as single-entity transfection and To probe the mechanism of SNA action, we have chosen gene silencing agents and have been used as lead therapeutic Drosophila as a model system since it provides the ability to ge- constructs in several disease models. However, the manner in which netically and biochemically manipulate the RNAi pathway (19). modified siRNA duplex strands that comprise the SNA lead to gene Moreover, the Drosophila RNAi pathway is well understood and is silencing is not understood. Herein, a systematic analysis of siRNA virtually identical to the mammalian pathway (20). It begins with biochemistry involving SNAs shows that Dicer cleaves the modified the introduction of double-stranded RNA (dsRNA) into cells. siRNA duplex from the surface of the nanoparticle, and the liberated When dsRNA is detected in the cytoplasm, it is processed into 22- siRNA subsequently functions in a way that is dependent on the nucleotide duplex siRNAs by the ribonuclease Dicer-2 (Dcr-2), canonical RNA interference mechanism. By leveraging this under- which is in complex form with Loquacious (Loqs)—a Drosophila standing, a class of SNAs was chemically designed which increases ortholog of TRBP (19, 21). Each duplex siRNA product is then the siRNA content by an order of magnitude through covalent released, and thereafter the siRNA duplex binds to a complex attachment of each strand of the duplex. As a consequence of in- composed of Dcr-2 and R2D2, another TRBP ortholog. This creased nucleic acid content, this nanostructure architecture exhibits forms the R2D2-Dcr-2 Initiator (RDI) complex (22, 23). The RDI CHEMISTRY less cell cytotoxicity than conventional SNAs without a decrease in complex then recruits a Taf11 protein tetramer to form the RNA- siRNA activity. induced silencing complex (RISC) Loading Complex (RLC) (22, 24). The duplex siRNA then transfers its binding from RLC to the spherical nucleic acids | siRNA processing | gene regulation protein Argonaute 2 (Ago2), creating the pre-RISC complex composed of siRNA duplex and Ago2 (25). Ago2 carries out mall interfering RNAs (siRNAs) are 22-nucleotide-long specific cleavage of the passenger strand of the duplex siRNA, and SRNAs that are broadly utilized agents for gene silencing in only the guide strand is retained with Ago2 to form the mature BIOCHEMISTRY biomedical research and disease treatment. Silencing of disease- RISC complex (25–27). RISC is the effector for RNAi by cleaving causing genes by RNA interference (RNAi) offers the ability to target genetic diseases that cannot be regulated with small- Significance molecule drugs, estimated to be 85 to 90% of the protein-coding genome (1). Additionally, since the physicochemical properties Although small interfering RNAs (siRNAs) are promising agents of small RNAs are largely sequence-independent, siRNA ther- for treating many diseases, cellular delivery difficulties have apeutics, in principle, should enable the more rapid development prevented their widespread application. The use of spherical of drugs. However, to be effective as drugs, delivery issues in- nucleic acids (SNAs) as single-entity gene regulation agents volving siRNAs must be overcome (2). Specifically, one must be could overcome this limitation, since SNAs naturally and rap- able to deliver the siRNA in active form to the tissues and cells idly enter over 50 cell types. Consequently, siRNA-SNAs have that matter. Since siRNAs, when systemically injected, distribute been extensively used for effecting knockdown in both re- predominately to the liver and spleen and are rapidly degraded search and clinical settings. However, the mechanism of gene in serum, RNAi drug development still faces challenges (3). regulation by siRNA-SNAs is not well understood. Herein, we To overcome these problems, we have explored the use of study cytosolic processing of siRNA-SNAs and how it leads to spherical nucleic acids (SNAs) as therapeutic modalities. SNAs gene knockdown. Informed by this mechanism, we designed typically comprise a spherical nanoparticle densely functional- an SNA architecture with an order-of-magnitude-higher siRNA ized with highly oriented oligonucleotides (4–7). Remarkably, density. Importantly, this higher density reduces the cellular SNAs, unlike linear nucleic acids, are rapidly and actively in- toxicity of SNAs without loss in RNA interference performance. ternalized by a wide variety of mammalian cells, and the unusual architecture inhibits oligonucleotide degradation (8–12). SNAs Author contributions: G.Y., D.M.P., R.W.C., and C.A.M. designed research; G.Y., R.J.S., have been used to treat many disease conditions in the clinic, D.M.P., and J.Q.N. performed research; G.Y. and R.J.S. contributed new reagents/analytic tools; G.Y., R.J.S., and R.W.C. analyzed data; and G.Y., R.W.C., and C.A.M. wrote including glioblastoma multiforme, psoriasis, and a variety of the paper. – cancers (13 18). In addition, they have shown promise in mouse Reviewers: D.G.A., Massachusetts Institute of Technology; and E.J.S., University of Mas- models for treating diabetic ulcers and spinal muscular atrophy. sachusetts Medical School. The prototypical SNA involves a gold nanoparticle chemically The authors declare no competing interest. modified with thiol-terminated siRNA duplexes. In this archi- Published under the PNAS license. tecture, the siRNA sense (passenger) strand is covalently anchored 1To whom correspondence may be addressed. Email: [email protected] or to the gold surface through thiol adsorption, and the antisense [email protected]. (guide) strand is noncovalently base-paired to the passenger strand. This article contains supporting information online at https://www.pnas.org/lookup/suppl/ Although these structures can function as potent inhibitors of gene doi:10.1073/pnas.1915907117/-/DCSupplemental. expression, no one has probed the mechanism of action of these www.pnas.org/cgi/doi/10.1073/pnas.1915907117 PNAS Latest Articles | 1of9 Downloaded by guest on September 27, 2021 any messenger RNA (mRNA) that anneals to the siRNA guide guide strand after unwinding. 3) Ago2 directly binds to the strand. A simplified version of this pathway is shown in Fig. 1A modified siRNA duplexes on the nanoparticle, and Ago2 is re- (blue shaded region). leased with the guide strand after unwinding. 4) Dcr-2/Loqs Since SNAs cannot be clearly categorized as containing either complexes bind to the modified siRNA duplexes on the nano- dsRNA or bona fide duplex siRNA, we considered 5 possible particle and cleave the siRNA, and the resulting duplex siRNAs mechanisms for explaining how SNAs silence gene expression are released for entry into the pathway as RDI complexes. 5) (Fig. 1A): 1) Modified siRNA duplexes on the nanoparticle The SNAs use a mechanism independent of RNAi. surface are passively released through the desorption of the gold- Here, we describe a set of experiments that evaluate these thiolate bond followed by entry into the RNAi pathway as RDI possibilities, and from them we have determined that the fourth complexes. 2) RDI, RLC, and pre-RISC complexes serially as- pathway (Dcr-2 cleavage of siRNA from the nanoparticle) ac- semble on the nanoparticle, and active RISC is released with the counts for how SNAs silence gene expression. Importantly, an AB150 *** *** dsRNA 100 ns 50 Relative Expression (%) 0 Buffer Duplex Duplex Cleavage Nontargeting SNA Cleavage Targeting SNA siRNA 4 Linear siRNA 1 Desorption 3 SNA Passenger 2 Strand Cleavage Minimal RISC Passenger Legend Strand Cleavage Guide Strand Passenger Dicer-2 R2D2 Strand Passenger Strand Cleavage Linker Loqs Ago2 Holo-RISC Fig. 1. The RNAi pathway in Drosophila and possible mechanisms for SNA processing. (A) The blue shade marks the canonical RNAi pathway in Drosophila: Dicer-2 and Loqs bind to long dsRNA and cleave it into a 22-nucleotide duplex siRNA. Dicer-2 and R2D2 bind to this siRNA and form the RDI complex. Then Ago2 is recruited, which cleaves the passenger strand, forming active RISC. Pathway 1 is characterized by the desorption of the siRNA from the surface of the SNA, which then behaves like free linear siRNA in its fate after desorption. Pathway 2 initiates with a Dicer-2/R2D2 complex binding to siRNA on the surface of the SNA to form a bound RDI complex. It then recruits Ago2 to the SNA, which forms RISC with the guide strand and is free to dissociate. Pathway 3 is characterized by Ago2 directly binding and processing the siRNA on the surface of the SNA and forms a “minimal RISC.” Finally, pathway 4 initiates with a Dicer-2/Loqs complex cleaving the SNA-bound siRNA, and the liberated siRNA then enters the canonical RNAi pathway. Common entry points into the pathway are shown in dashed boxes, while the catalytically active endpoints are shown in solid boxes. Solid and dashed arrows depict experimentally verified and hypothesized steps, respectively. Shading illustrates steps that involve linear (blue) or spherical (green) forms of RNA.
Details
-
File Typepdf
-
Upload Time-
-
Content LanguagesEnglish
-
Upload UserAnonymous/Not logged-in
-
File Pages9 Page
-
File Size-