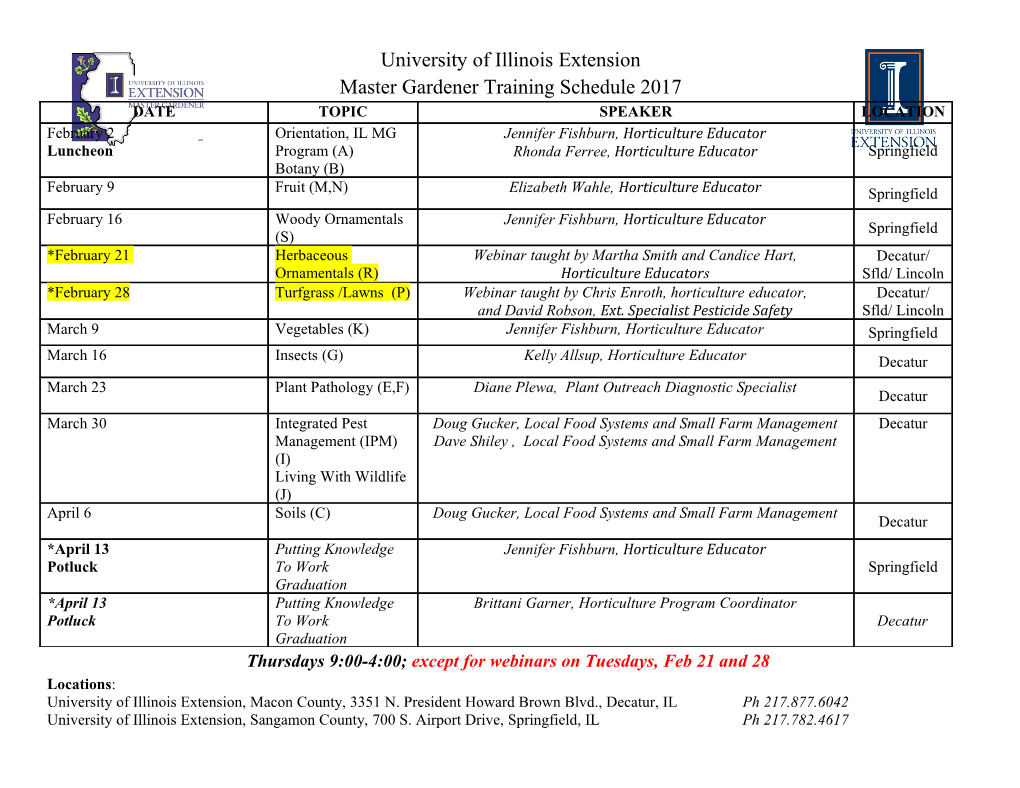
bioRxiv preprint doi: https://doi.org/10.1101/2020.03.15.992164; this version posted October 26, 2020. The copyright holder for this preprint (which was not certified by peer review) is the author/funder. All rights reserved. No reuse allowed without permission. Functional Organization of the Maternal and Paternal Human 4D Nucleome Stephen Lindsly1, Wenlong Jia2, Haiming Chen1, Sijia Liu3, Scott Ronquist1, Can Chen4;7, Xingzhao Wen5, Gabrielle A. Dotson1, Charles Ryan1;8;9 Gilbert S. Omenn1, Shuai Cheng Li2, Max Wicha1;6, Alnawaz Rehemtulla6, Lindsey Muir1, Indika Rajapakse1;7;∗ 1Department of Computational Medicine and Bioinformatics, University of Michigan, Ann Arbor 2Department of Computer Science, City University of Hong Kong 3MIT-IBM Watson AI Lab, IBM Research 4Department of Electrical Engineering and Computer Science, University of Michigan, Ann Arbor 5Department of Bioengineering, University of California San Diego, La Jolla 6Department of Hematology/Oncology, University of Michigan, Ann Arbor 7Department of Mathematics, University of Michigan, Ann Arbor 8Medical Scientist Training Program, University of Michigan, Ann Arbor 9Program in Cellular and Molecular Biology, University of Michigan, Ann Arbor ∗To whom correspondence should be addressed; E-mail: [email protected]. bioRxiv preprint doi: https://doi.org/10.1101/2020.03.15.992164; this version posted October 26, 2020. The copyright holder for this preprint (which was not certified by peer review) is the author/funder. All rights reserved. No reuse allowed without permission. Abstract Every human somatic cell inherits a maternal and paternal genome, which work together to give rise to cellular phenotype. However, the allele-specific re- lationship between gene expression and genome structure through the cell cy- cle is largely unknown. By integrating haplotype-resolved genome-wide chro- mosome conformation capture, mature and nascent mRNA, and protein bind- ing, we investigate this relationship both globally and locally. We introduce the maternal and paternal 4DN, enabling detailed analysis of the mechanisms and dynamics of genome structure and gene function for diploid organisms. Our analyses find significant coordination between allelic expression biases and lo- cal genome conformation, and notably absent expression bias in universally essential cell cycle and glycolysis genes. We offer a model in which coordinated biallelic expression reflects prioritized preservation of essential gene sets. bioRxiv preprint doi: https://doi.org/10.1101/2020.03.15.992164; this version posted October 26, 2020. The copyright holder for this preprint (which was not certified by peer review) is the author/funder. All rights reserved. No reuse allowed without permission. Introduction The redundancy inherent in the diploid genome makes it robust to potentially harmful muta- tions. Dependence on a single allele, for example in genes with monoallelic or allele biased expression (MAE, ABE), may increase vulnerability to disease. In familial cancer syndromes, individuals who inherit genetic loss-of-function mutations have a single functional allele, re- ferred to as loss of heterozygosity (LOH) (1). BRCA1 and BRCA2 are quintessential examples, where LOH leads to a significant increase in the likelihood of developing breast cancer (2, 3). Imprinted genes are also associated with multiple disease phenotypes such as Angelman and Prader-Willi syndromes (4, 5). This raises the possibility that other MAE or ABE genes are be associated with disease, but the contribution of allelic bias to disease phenotypes remains poorly understood. A major step towards understanding the contribution of allelic bias to disease will be to identify ABE genes and gene modules, recognizing that functionally important biases may be transient and challenging to detect. Differences in allelic expression may result from single nu- cleotide variants (SNVs), insertions, deletions (InDels), and chromatin modifications (6–10). By some estimates, ABE occurs in 4-26% of expressed genes across tissues and individu- als (11). In addition, higher order chromatin conformation and spatial positioning in the nucleus shape gene expression (12–15). This may lead to allele-specific differences as the maternal and paternal alleles have been shown to be distant in the nucleus through techniques such as fluo- rescent in situ hybridization (FISH) (16, 17). Allele-specific expression and 3D structures are frequently not distinguished in genomics methods such as RNA-sequencing and Hi-C (genome- wide chromosome conformation capture), complicating interpretation of structure-function re- lationships. However, complete phasing of the two genomes remains a significant challenge in the field. To uncover ABE and its relationship to genome structure over time, we integrated allele- specific RNA-seq, Bru-seq, and Hi-C across three phases of the cell cycle in B-lymphocytes (GM12878, Figure 1). The RNA-seq and Bru-seq data were separated into their maternal bioRxiv preprint doi: https://doi.org/10.1101/2020.03.15.992164; this version posted October 26, 2020. The copyright holder for this preprint (which was not certified by peer review) is the author/funder. All rights reserved. No reuse allowed without permission. and paternal components through SNVs/InDels. The Hi-C data was separated using our novel phasing algorithm, HaploHiC, which utilizes SNVs/InDels to impute Hi-C reads of unknown parental origin. We incorporated publicly available allele-specific protein binding data (ChIP- seq) to better understand the mechanisms behind the gene expression and genome structure re- lationship (7,18). We performed a genome-wide investigation for gene expression biases, chro- matin compartment switching, and topologically associated domain (TAD) boundary changes between alleles and cell cycle phases. Along with known XCI and imprinted genes, we found notable patterns of ABE across the cell cycle for hundreds of genes that are not well ap- preciated in current literature, and corresponding allelic biases in protein binding at many of these loci. We found that differentially expressed genes were significantly more likely to have changes in their local genomic structure than random allele-specific genes. Additionally, we observed a pronounced lack of ABE in crucial biological pathways and essential genes. Our in- tegrated analysis represents an allele-specific extension of the 4D Nucleome (4DN), which de- scribes the relationship between genome structure, gene expression, and cell phenotype through time (19–21). Taken together, our findings demonstrate the value of analyzing ABE in the con- text of corresponding structural and epigenetic alterations across the cell cycle. This approach will be critical in future investigations of human phenotypic traits and their penetrance, genetic diseases, vulnerability to complex disorders, and tumorigenesis. bioRxiv preprint doi: https://doi.org/10.1101/2020.03.15.992164; this version posted October 26, 2020. The copyright holder for this preprint (which was not certified by peer review) is the author/funder. All rights reserved. No reuse allowed without permission. Fig. 1: Experimental and allelic separation workflow. Cell cycle sorted cells were extracted for RNA-seq, Bru-seq, and Hi-C (left to right, respectively). RNA-seq and Bru-seq data were allelically phased via SNVs/InDels (left). SNV/InDel based imputation and haplotype phasing of Hi-C data using HaploHiC (right). These data provide quantitative measures of structure and function of the maternal and paternal genomes through the cell cycle. bioRxiv preprint doi: https://doi.org/10.1101/2020.03.15.992164; this version posted October 26, 2020. The copyright holder for this preprint (which was not certified by peer review) is the author/funder. All rights reserved. No reuse allowed without permission. Results Chromosome-Scale Maternal and Paternal Differences Spatial positioning of genes within the nucleus is known to be associated with transcriptional status (12–15). One might expect that the maternal and paternal copies of each chromosome would stay close together to ensure that their respective alleles have equal opportunities for tran- scription. Imaging of chromosome territories has shown that this is often not the case (Figure 2A, Supplementary Notes C)(22–24). This inspired us to investigate whether the two genomes operate in a symmetric fashion, or if allele-specific differences exist between the genomes re- garding their respective chromatin organization patterns and gene expression profiles. We an- alyzed parentally phased whole-chromosome Hi-C and RNA-seq data at 1 Mb resolution to identify allele-specific differences in both chromosome structure and function, respectively. We subtracted each chromosome’s paternal Hi-C matrix from the maternal matrix and found the Frobenius norm of the resulting difference matrix. The Frobenius norm here provides a mea- sure of distance between matrices, where equivalent parental genome structures would result in a value of zero. Similarly, we subtracted the phased RNA-seq vectors in log2 scale and found the Frobenius norm of each difference vector. The Frobenius norms were adjusted for chromosome size and normalized for both Hi-C and RNA-seq. We found that all chromosomes have allelic differences in both structure (Hi-C, blue) and function (RNA-seq, red) (Figure 2B). Chromosome X had the largest structural difference, as expected, followed by Chromosomes 9, 21, and 14. Chromosome X had the most extreme functional difference as well, followed
Details
-
File Typepdf
-
Upload Time-
-
Content LanguagesEnglish
-
Upload UserAnonymous/Not logged-in
-
File Pages62 Page
-
File Size-