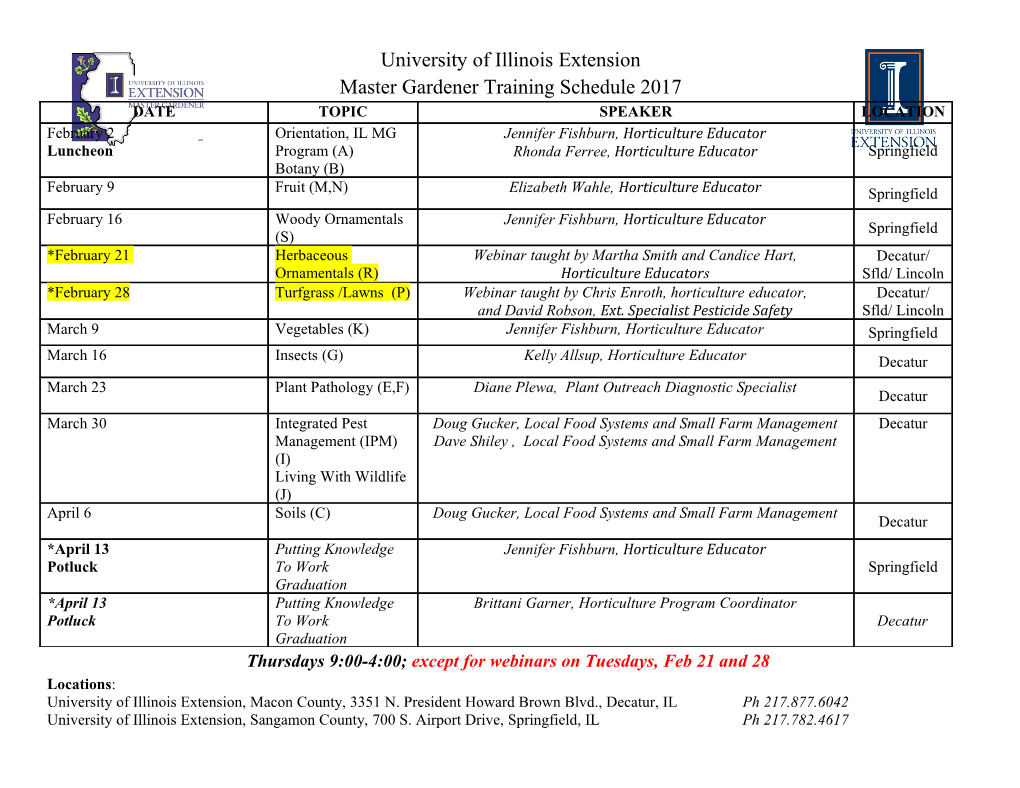
1 Premature Polyadenylation of MAGI3 Produces a Dominantly-Acting Oncogene in Human 2 Breast Cancer 3 4 5 Thomas K. Ni1,2,* and Charlotte Kuperwasser1,2,* 6 7 8 Affiliations: 9 1 Department of Developmental, Chemical and Molecular Biology, Tufts University, 150 10 Harrison Ave, Boston, MA 02111, USA 11 2 Molecular Oncology Research Institute, Tufts Medical Center, 800 Washington St, Boston, MA 12 02111, USA 13 * Correspondence to: 14 [email protected] 15 [email protected] 16 17 18 Competing Interests Statement: 19 The authors declare that there are no conflicts of interest. 1 20 Abstract 21 Genetic mutation, chromosomal rearrangement and copy number amplification are common 22 mechanisms responsible for generating gain-of-function, cancer-causing alterations. Here we 23 report a new mechanism by which premature cleavage and polyadenylation (pPA) of RNA can 24 produce an oncogenic protein. We identify a pPA event at a cryptic intronic poly(A) signal in 25 MAGI3, occurring in the absence of local exonic and intronic mutations. The altered mRNA 26 isoform, called MAGI3pPA, produces a truncated protein that acts in a dominant-negative manner 27 to prevent full-length MAGI3 from interacting with the YAP oncoprotein, thereby relieving YAP 28 inhibition and promoting malignant transformation of human mammary epithelial cells. We 29 additionally find evidence for recurrent expression of MAGI3pPA in primary human breast tumors 30 but not in tumor-adjacent normal tissues. Our results provide an example of how pPA contributes 31 to cancer by generating a truncated mRNA isoform that encodes an oncogenic, gain-of-function 32 protein. 2 33 Introduction 34 Genetic mutation, copy number gain/loss and chromosomal rearrangement are major 35 mechanisms of genome alteration that underlie cancer pathogenesis. Frequent alteration of a 36 gene by one or more of these mechanisms represents prima facie evidence for its role as a driver 37 – a gene whose alterations are causative in cancer. As such, within the last decade, tremendous 38 efforts have focused on the systematic, genome-wide surveys for these events in cancer. 39 Interestingly, results from these analyses indicate that many tumors harbor alterations in only 1-2 40 known drivers, and ~15% of tumors lack alterations in even a single known driver (Imielinski et 41 al. 2012; Garraway and Lander 2013). Since solid tumors arise due to oncogenic cooperation 42 between alterations in multiple drivers (Fearon and Vogelstein 1990; Hanahan and Weinberg 43 2000), a significant number of cancer-relevant genes are currently being missed by genomic 44 analyses because so many genes are ostensibly altered at frequencies below the threshold for 45 driver detection (Lawrence et al. 2014). 46 While it has been posited that unidentified drivers are difficult to identify because they 47 are infrequently altered in cancer, it is equally plausible that additional mechanisms, beyond 48 those regularly interrogated by current genomics platforms, may be responsible for generating 49 driver alterations. In fact, as new mechanisms of alteration relevant to cancer are discovered, a 50 number of “infrequently altered” genes are reclassified as frequently altered. For example, the 51 discovery of promoter mutations in the gene encoding telomerase has resulted in the 52 reclassification of TERT as a frequently altered melanoma driver (Huang et al. 2013; Horn et al. 53 2013). Therefore, identifying cancer-specific alterations generated by previously unappreciated 54 mechanisms represents a fundamentally important prerequisite for driver gene identification. 3 55 Ni et al. previously identified several dozen candidate cancer driver genes from a forward 56 genetics screen for tumorigenesis in mice (Ni et al. 2013). Despite being functionally implicated 57 in tumorigenesis, some of these candidate drivers were rarely mutated, amplified or deleted in 58 human cancer. This incongruence led us to hypothesize that some of these candidate drivers may 59 appear to be infrequently altered because their driver alterations may be caused by mechanisms 60 that are not regularly interrogated. Here, we report that one of these candidate drivers, MAGI3, is 61 indeed recurrently altered in breast cancer by premature cleavage and polyadenylation (pPA), a 62 mechanism not widely appreciated for its involvement in cancer, and the resulting truncation of 63 the MAGI3 gene products functionally contributes to malignant transformation. 64 65 Results 66 A Premature Polyadenylation Event Generates a Truncated MAGI3 Protein in MDA-MB- 67 231 Breast Cancer Cells 68 Many mechanisms are involved in conveying genetic information from genes to their 69 mRNA and protein products. When gone awry, any of these mechanisms could alter cancer- 70 relevant genes or their gene products. To investigate our hypothesis that some candidate drivers 71 may be altered by underlying mechanisms not widely thought to be involved cancer, we 72 considered strategies capable of broadly capturing many types of alteration events. While there is 73 no standard strategy for this purpose, we reasoned that changes in genetic information, in most 74 cases, must ultimately manifest at the protein level in order to contribute to cancer. Thus, our 75 strategy focused on identifying novel, unannotated protein products of a limited number of genes 76 that we suspected to be involved in cancer due to their previous identification by a forward 77 genetics screen for tumorigenesis in mice (Ni et al. 2013). We focused specifically on products 4 78 exhibiting detectable size differences from wild-type protein isoforms since these may be more 79 likely to cause significant functional effects. Once such a product was identified, we would 80 determine whether the altered protein is generated by a genetic mutation in the coding regions of 81 the corresponding gene (Figure 1A). If not due to coding region genetic mutation, we would 82 attempt to identify the alteration mechanism, establish recurrence for the specific alteration event 83 in cancer and investigate its functional importance in malignant transformation. 84 Accordingly, we interrogated breast cancer cell lines for evidence of previously 85 unannotated protein products of candidate drivers. Immunoblotting across this cell line panel for 86 one such candidate, MAGI3, revealed a number of extra bands in addition to the two full-length 87 protein isoforms, α and β (Figure 1B). We found that one of these bands, faintly appearing in 88 most of the cell lines, was non-specific to MAGI3 since it could not be depleted by multiple 89 shRNA targeting MAGI3 (Figure 1C). However, in MDA-MB-231 breast cancer cells, a strong 90 band of lower molecular weight was observed and could be specifically depleted by RNAi 91 (Figures 1B and 1C). Notably, this truncation was not expressed in the non-transformed 92 MCF10A mammary cell line or TERT-immortalized human mammary epithelial cells (HMEC) 93 (Figures 1B and 1D). 94 MAGI3 is a scaffolding protein with 2 WW and 6 PDZ domains, along with a 95 catalytically inactive region of homology to the yeast guanylate kinase (Figure 1E) (Funke, 96 Dakoji, and Bredt 2005). Mass spectrometry (MS) analysis of the immunoprecipitated truncation 97 mapped many peptides corresponding to the N-terminal half of MAGI3, but detected no peptides 98 from the PDZ domains 3-6 that consist of the C-terminal half of MAGI3 (Figure 1F). To identify 99 the genetic alteration responsible for this truncation, we sequenced all 22 exons and flanking 100 intron sequences of MAGI3 but found no mutations. This indicated that the truncation is not 5 101 generated by DNA mutation of the MAGI3 coding sequence or splice sites. We subsequently 102 used 3′ rapid amplification of cDNA ends (RACE) to isolate the transcript responsible for the 103 truncated MAGI3 protein. This yielded a truncated MAGI3 mRNA isoform corresponding to the 104 size and mapped regions of the truncated protein (Figure 1G). Sequencing the 3′ end of the 105 mRNA revealed a cleavage and polyadenylation event involving a cryptic poly(A) signal (PAS) 106 located in MAGI3 intron 10 (Figure 1H and Figure 1—figure supplement 1). Consequently, the 107 normal splicing event from exon 10 to exon 11 of MAGI3 does not occur in the truncated mRNA 108 (MAGI3pPA), resulting in a stop codon several nucleotides into intron 10 and premature 109 transcription termination following the cryptic intronic PAS (Figure 1H). 110 Local cis-acting DNA mutations affecting upstream U1 snRNA binding sites or 111 downstream U-rich elements (URE) can affect splicing as well as cleavage and polyadenylation 112 (Kaida et al. 2010; Berg et al. 2012; Almada et al. 2013; Proudfoot 2011). Therefore, we 113 sequenced the entire MAGI3 intron 10 from MDA-MB-231 cells but were unable to find any 114 mutations (Figure 1—figure supplement 1). We also sequenced the entire region of the 115 MAGI3pPA 3′ RACE product corresponding to intron 10 and the flanking exon 10 sequence, but 116 again uncovered no mutations (Figure 1—figure supplement 2). Notably, the known cis-acting 117 sequences involved in proper splicing and repression of pPA, including the 118 upstream/downstream splice donors, upstream U1 snRNA binding site and downstream URE, 119 were intact in the sequencing analyses (Figure 1H and Figure 1—figure supplements 1 and 2). 120 We thus conclude that this pPA event in intron 10 of MAGI3 arises independently of local 121 genetic mutations. However, we cannot exclude the possibility that a long-range, non-coding 122 mutation in MAGI3 might play a role in this phenomenon. 123 6 124 Evidence for Recurrent Generation of MAGI3pPA in Human Breast Cancer 125 To determine whether the pPA product of MAGI3 is physiologically relevant in the 126 context of primary human breast tumors, we analyzed whole transcriptome shotgun sequencing 127 (RNA-Seq) data made available by The Cancer Genome Atlas (TCGA) and a previously 128 published RNA-Seq study (Varley et al.
Details
-
File Typepdf
-
Upload Time-
-
Content LanguagesEnglish
-
Upload UserAnonymous/Not logged-in
-
File Pages46 Page
-
File Size-