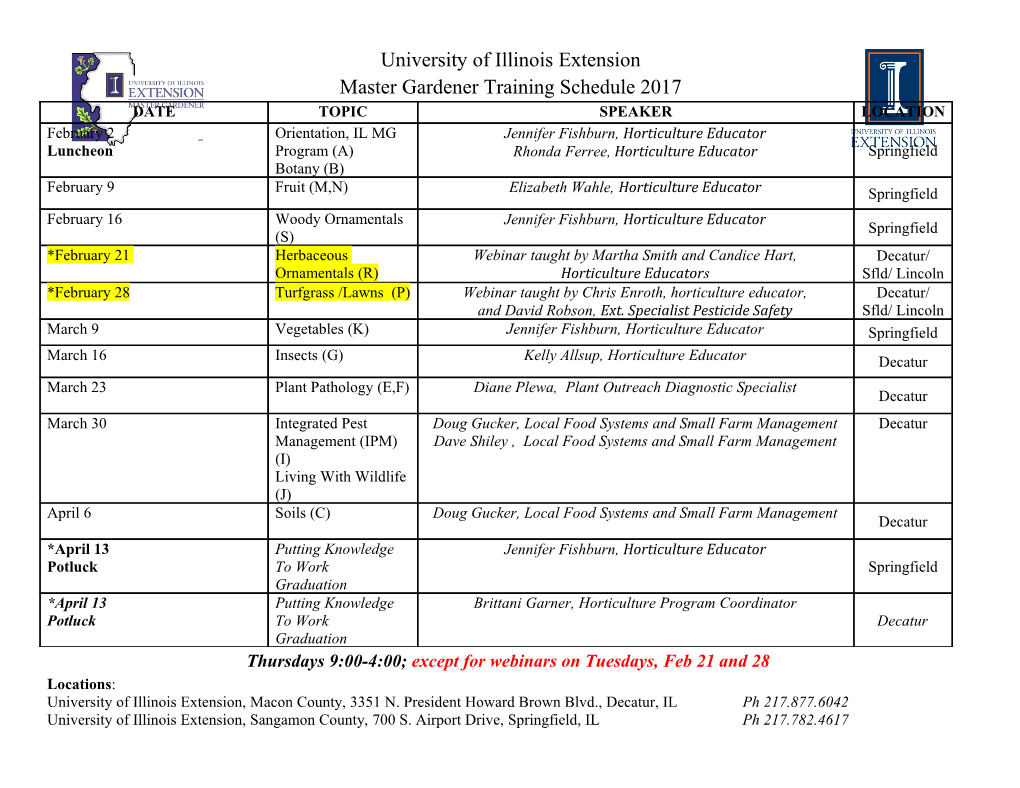
572. BIOCHEMISTRY: MORTLOCK ET AL. PROC. N. A. S. 5 Dische, Z., "Color reaction of nucleic acid components," in The Nucleic Acids, ed. E. Chargaff and J. Davidson (New York: Academic Press, 1955), Vol. 1, pp. 258-303. 6 Burton, K., Biochem. J., 62, 315 (1956). 7Oyama, W. I., and H. Eagle, Proc. Soc. Exptl. Biol. Med., 91, 305 (1956). 8 Marmur, J., J. Mol. Biol., 3, 208 (1961). 9 Tamm, C., M. E. Hoder, and E. Chargaff, J. Bio. Chem., 195, 49 (1952). '0Szybalski, W., Anal. Biochem., 3, 267 (1962). 11 Sueoka, N., J. Mol. Biol., 3, 31 (1961). 12Trowne, P. W., and B. R. Rabin, these PROCEEDINGS, 52, 88 (1964). 13Goldberg, I. H., M. Rabinowitz, and E. Reich, these PROCEEDINGS, 48, 2094 (1962). 14 Siminoff, P., Appl. Microbiol., 9, 66 (1961). 15 Crowther, D., and J. Melnick, Virology, 15, 65 (1964). I6 Smith, C. G., W. L. Lummis, and J. E. Grady, Cancer Res., 19, 847 (1959). 17 Reich, E., Science, 143, 684 (1964). 18 These experiments were performed by Drs. J. J. Vavra and H. E. Renis of The Upjohn Company, and by Dr. J. J. Holland, University of California, Redwood, California. 19 Liersch, M., and G. Hartman, Biochem. Z., 340, 390 (1964). 20 DiMarco, A., et al., Nature, 201, 707 (1964). 21 Ward, D., and E. Reich, Federation Proc., 24, 603 (1965). 22 Chamberlin, M., and P. Berg, these PROCEEDINGS, 48, 81 (1962). 23 Goldberg, I. H., Biochim. Biophys. Acta, 51, 201 (1961). 24 Magee, W. E., Virology, 17, 604 (1962). A BASIS FOR UTILIZATION OF UNNATURAL PENTOSES AND PENTJTOLS BY AEROBACTER AEROGENES* BY R. P. MORTLOCK,t D. D. Fossirr, AND W. A. WOOD DEPARTMENT OF BIOCHEMISTRY, MICHIGAN STATE UNIVERSITY, EAST LANSING, MICHIGAN Communicated by H. G. Wood, June 18, 1966 Although not rigorously studied, it has long appeared that bacteria are unique in their ability to metabolize those organic materials which are rarely, if ever, found in nature. Aerobacter aerogenes, PRL R3, particularly illustrates this phenomenon with its ability to utilize as a source of energy seven of the eight aldopentoses and all four of the pentitols.' 2 Of this group of 11 structures, D-ribose, D-xylose, L- arabinose, D-arabitol, and ribitol are found in nature, whereas D-arabinose, D- lyxose, L-xylose, L-lyxose, xylitol, and L-arabitol3 seem to be rarely, if ever, en- countered in the natural environment.4 The routes of utilization of all of these C, compounds have been documented (Fig. 1).2. 5-18 Referring to Figure 1, it can be seen that metabolism of the unnatural compounds, D-lyxose and xylitol, for example, involves only one new or unique reaction for each, i.e., isomerization of D-lyxose or oxidation of xylitol to D-xylulose. The phosphorylation of D-xylulose is common to the route for utilization of D-xylose and D-arabitol, both naturally occurring substrates readily attacked by A. aero- genes. Similarly, the conversions of L-xylose, L-lyxose, and L-arabitol involve either isomerizations or an oxidation to L-xylulose.2 8 The subsequent reactions of the pathway are common to those for L-xylulose and L-ribulose2 19 which appear in nature as metabolic intermediates in utilization of L-ascorbate20 and L-arabi- Downloaded by guest on September 26, 2021 VOL. 54, 1965 BIOCHEMISTRY: MORTLOCK ET AL. 573 D-RIBOSE kinose D-RIBOSE-5-PHOSPHATE isomerose D-ARABID-ARABINOSENOSE - isomeroseIsmrs -D-RIBULOSED-RIBULOSE kinose D-RIBULOSE-5- PHOSPHATE RIBITOL dehydrogenose i D-XYLOSE isomerose 3-epimerose D-LYXOSE isomerose D-XYLULOSE kino, ID-XYLULOSE-5-PHOSPHATEI D-ARABITOL dehydrogenose XY LITOL dehydrogenose 4-epimerase -ARABINOSE 4r- L-RIBULOSE k L-RIBULOSE-5-PHOSPHATE isomerose L-XYLOSE - 3-epimerose L-LYXOSE isomerose L-XYLULOSE L-XYLULOSE-5-PHOSPHATE L-ARAB TOL dehydrogenose I FIG. 1.-Pathways of pentose and pentitol dissimilation in A. aerogenes. nose,'4 16, 18 respectively. Thus, only one unique reaction for each rare compound is necessary for its dissimilation. The remainder of the sequence involves inducible enzymes which function in dissimilation of naturally occurring pentoses and penti- tols and for which the necessary genes are pre-existent.2" 22 Thus, the central problem concerns the means by which the organism acquires an ability to catalyze the unique isomerizations and dehydrogenations which convert the rare structures to ketopentoses. From growth and induction experiments, it has been established that the necessary enzymes for conversion of the naturally occurring structures to D-xylulose-5-phosphate are formed in response to inducer in the usual way." 22 In addition, it is assumed that preservation of the genetic in- formation for these enzymes involves the usual selective forces. The same situation would not be predicted for the unnatural substrates. The existence of genes direct- ing the synthesis of unique enzymes seems unlikely since an environment for their selection presumably does not exist, and their eventual loss, if indeed they once existed, would result from mutations. Data indicating that a mutational event precedes the initiation of growth on the unnatural substrates have been obtained" 22 and, hence, it is possible that these mutations lead to the acquisition of a new enzyme. Yet this is unlikely because several specific acquisitions of a unique enzyme would be indicated from the ability to grow on several of the unnatural substrates. Further, because of the relatively short period required to select a mutant population for many of these substrates, each mutation leading to a new enzyme would have to occur at a very high fre- quency. Thus, these acquisitions of a unique activity must be viewed as short-term Downloaded by guest on September 26, 2021 574 BIOCHEMISTRY: MORTLOCK ET AL. PROC. N. A. S. events and not as evolutionary gain processes. Hence, the mutational acquisition of unique enzymes seems unlikely. It is the purpose of this report to present data which show, when considered with already published observations, that a-fortuitous combination of circumstances can act as a mechanism which will allow growth on several unnatural pentoses and pentitols without necessitating the acquisition of a new enzyme. Methods.-Aerobacter aerogenes was cultured at 300C on a salts-medium supple- mented with either 0.5 per cent carbohydrate, 1 per cent peptone, or casein hydroly- sate (Difco).' The method of Lin et al.23 was used to determine the proportion of constitutive cells and to isolate mutant strains, 4R1 and 5R1, after growth of the wild type on xylitol or L-arabitol, respectively. Preparation of extracts and anti- sera and methods of purification and determination of S20 values were described pre- viously.24 Pentitol dehydrogenases were assayed as the reduction of NAD by pentitol in the presence of semicarbazide buffer, pH 8.5,25 or by the oxidation of NADH by ketopentose in tris buffer, pH 7.5.24 One unit of dehydrogenase cata- lyzed an absorbancy change of 1.0 per minute at 340 mu in a reaction volume of 0.15 ml (1 = 1 cm). Isomerase activity was measured as the rate of ketopentose forma- tion by the method of Anderson and Wood.8 One unit of isomerase in 2.0 ml cata- lyzed the formation of 1 umole of ketopentose per hour at 370C. Protein was de- termined by the method of Lowry et al.,26 and ketopentose was estimated by the cysteine-carbozole test of Dische and Borenfreund.2Y Results.-Enzymes for xylitol and L-arabitol utilization: The data of Mortlock et al.24 28 show that the xylitol dehydrogenase activity of cells grown on xylitol is attributable to a ribitol dehydrogenase which is present in high levels. Both dehy- drogenase activities fractionate identically on DEAE cellulose and in classical pro- cedures; each has the same S20 value (6.0-6.2), and antibodies elicited by ribitol dehydrogenase purified from D-arabinose-grown cells inhibited xylitol dehydro- genase and ribitol dehydrogenase from xylitol-grown cells to the same extent (91- 96%). Careful examination of extracts by DEAE-cellulose chromatography, sucrose density centrifugation, sucrose density electrophoresis, and by disk electro- phoresis failed to reveal the existence of another xylitol dehydrogenase. Similarly, the L-arabitol dehydrogenase activity of cells grown on L-arabitol ap- pears to be attributable solely to ribitol dehydrogenase. The ratios of ribitol dehy- drogenase to L-arabitol dehydrogenase in the crude extract and various purified fractions were identical. Also, no other L-arabitol dehydrogenase was found in effluents from DEAE cellulose columns or by disk electrophoresis. We have now crystallized (a) the inducible ribitol dehydrogenase from ribitol- grown cells, (b) the ribitol dehydrogenase from a constitutive mutant isolated after growth on xylitol, and (c) the ribitol dehydrogenase isolated from a constitutive mutant isolated after growth on L-arabitol.29 Each catalyzes the following reac- tions: Ribitol + DPN+ >r± D-ribulose + DPNH + H+ Xylitol + DPN+ T± D-xylulose + DPNH + H+ L-arabitol + DPN+ 2± L-xylulose + DPNH + H+ As shown in Table 1, the specific activities of the crystalline material were identical in each case (32,000). The relative maximum velocities for xylitol and L-arabitol Downloaded by guest on September 26, 2021 VOL. 54,1965 BIOCHEMISTRY: MORTLOCK ET AL. 575 TABLE I COMPARISON OF RIBITOL D)EHYDROGENASE FROM DIFFERENT SOURCES Dehydrogenasec Inducible, Constitutive mutant, Constitutive mutant, ribitol culture xylitol culture L-arabitol culture KM, ribitol 2.6 X 10-' 3.5 X 10-3 2.6 X 10-3 L-arabitol 2.9 X 10-1 2.5 X 10-1 4.0 X 10-1 Xylitol 2.9 X 10-1 2.7 X 10-1 4.2 X 10-1 Ratio-V.., ribitol 100 100 100 L-arabitol 35 39 46 Xylitol 34 23 44 Heat sensitivity 4.5-4.8 5.0 5.0 S20,.
Details
-
File Typepdf
-
Upload Time-
-
Content LanguagesEnglish
-
Upload UserAnonymous/Not logged-in
-
File Pages8 Page
-
File Size-