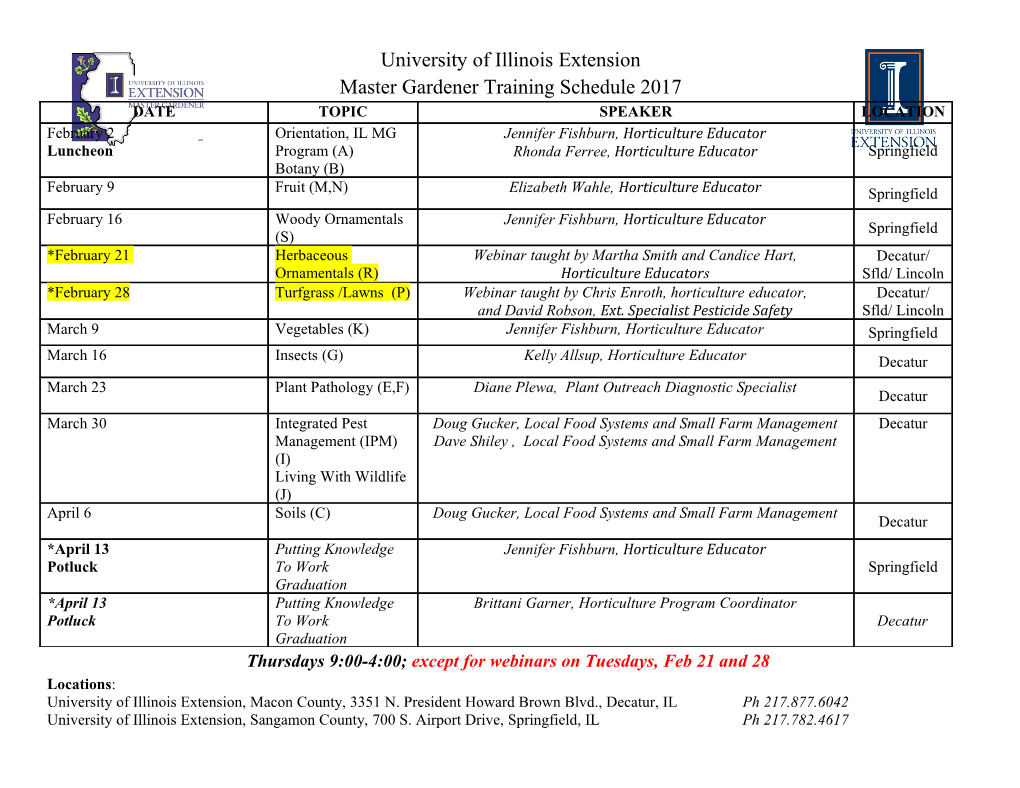
Low-temperature, manganese oxide-based, thermochemical water splitting cycle Bingjun Xu, Yashodhan Bhawe, and Mark E. Davis1 Chemical Engineering, California Institute of Technology, Pasadena, CA 91125 Contributed by Mark E. Davis, April 17, 2012 (sent for review April 5, 2012) Thermochemical cycles that split water into stoichiometric amounts advantage of both the low-temperature multistep and high-tem- of hydrogen and oxygen below 1,000 °C, and do not involve toxic perature two-step cycles. Here, we show that more than two or corrosive intermediates, are highly desirable because they can reactions will be necessary to perform themochemical water split- convert heat into chemical energy in the form of hydrogen. We ting below 1,000 °C, and then introduce a new thermochemical report a manganese-based thermochemical cycle with a highest water splitting cycle that involves non-corrosive solids that can operating temperature of 850 °C that is completely recyclable and operate with a maximum temperature of 850 °C. does not involve toxic or corrosive components. The thermochemi- cal cycle utilizes redox reactions of Mn(II)/Mn(III) oxides. The shut- Results and Discussion tling of Naþ into and out of the manganese oxides in the hydrogen Thermodynamic Analysis of Two-Step Thermochemical Cycles Shows and oxygen evolution steps, respectively, provides the key thermo- Temperatures Above 1,000 °C will be Necessary. Consider a thermo- dynamic driving forces and allows for the cycle to be closed at tem- chemical cycle with the following two steps: (i) oxidation of a peratures below 1,000 °C. The production of hydrogen and oxygen metal or metal oxide, referred to as Red, by water to its oxidized is fully reproducible for at least five cycles. state, referred to as Ox, with the metal at a higher oxidation state; and (ii) thermal reduction of the Ox phase back to the Red phase, hydrogen production ∣ Na+ extraction ∣ multistep cycle accompanied by the release of oxygen, as follows: T1 Red þ H2O ¼ Ox þ H2 [1] hermochemical production of hydrogen and oxygen from Twater involves a series of chemical reactions that convert Ox ¼ Red þ 0 5 : [2] T2 . O2 water into stoichiometric amounts of hydrogen and oxygen using heat as the only energy source. Thermochemical water splitting is T1 and T2 are the reaction temperatures where ΔG ¼ 0 for of interest because it directly converts thermal energy into stored Eqs. 1 and 2, respectively. From the changes in the Gibbs free chemical energy (hydrogen and oxygen). Research on thermoche- energy, ΔGr ¼ ΔHr − TΔSr, expressions relating T1 to T2 with mical water splitting cycles largely began in the 1960s and 1970s 0 0 0 0 either (ΔH − ΔH )or(ΔS − ΔS ) held constant and involved nuclear reactors (1, 2) and solar collectors (3) as the f;Red f;Ox f;Red f;Ox (isenthalpic or isentropic, respectively) can be obtained (Fig. 1 energy sources. Numerous reviews of the thermochemical cycles and SI Appendix). proposed and experimentally investigated are available, e.g., (4). The thermodynamic data shown in Fig. 1 demonstrate that it A large number of thermochemical cycles for splitting water has is unlikely, if not impossible, to split water with a two-step cycle been proposed, and generally can be grouped into two broad where there is complete conversion between the oxidized and categories: high-temperature two-step processes (5, 6) and low- reduced forms at below 1,000 °C. Typical differences in the for- temperature multistep processes (7, 8). One of us (M.E.D.) has mation enthalpy (ΔΔH) and entropy (ΔΔS) of the Red and Ox conducted previous research on low-temperature multistep pro- phases for metal/oxide systems (SI Appendix, Table S2) are found cesses in the 1970s (9). to be below 400 kJ∕mol and 50 J∕mol∕K, respectively (conser- Low-temperature multistep processes, typically with the a vative upper bounds). Therefore, only the region above both the highest operating temperature below 1,000 °C, allow for the use lower black (entropic) and green (enthalpic) lines in Fig. 1 (blue of a broader spectrum of heat sources, such as heat from nuclear shaded area) has practical relevance, as it is only in this region power plants, and hence have attracted considerable attention. that both thermodynamic criteria are satisfied. The target region The majority of existing low-temperature processes produces for low-temperature thermal water splitting (yellow shaded area, intermediates that can be complex, corrosive halide mixtures. T1,T2 < 1;000 °C) has no overlap with the practically accessible One of these processes, the sulfur-iodine cycle, has been studied region when using a two-step process. Additionally, existing two- extensively, and even piloted for implementation (8). This pro- step thermochemical water splitting cycles that have been re- cess produces strongly acidic mixtures of sulfuric and iodic acids ported previously all operate at above 1,000 °C (5, 6, 11, 12). The that create significant corrosion issues, but requires only one conclusion that can be derived from these results is that thermo- high-temperature step at ca. 850 °C. chemical water splitting cycles accomplished below 1,000 °C will Two-step processes typically involve simpler reactions and require more than two steps, and is consistent with the previous intermediates, e.g., solid metal oxides, than the low-temperature thermodynamic analysis of Meredig and Wolverton (14). multistep cycles. However, the temperatures required to close these types of cycles are well above 1,000 °C. Because of the re- Multistep, Thermochemical Cycle for Splitting Water Below 1,000 °C. quirement of high-temperature heat sources, these types of cycles Thermochemical cycle overview. We have developed a Mn-based, have been investigated for use with solar concentrators (10, 11). These cycles typically consist of one step that involves the oxida- tion of a metal [such as zinc (12)] or a metal oxide [such as iron Author contributions: B.X., Y.B., and M.E.D. designed research; B.X. and Y.B. performed (II) oxide (6, 13)] by water to produce hydrogen, and a subse- research; B.X., Y.B., and M.E.D. analyzed data; and B.X., Y.B., and M.E.D. wrote the paper. quent step to recover the starting material from its oxidized form The authors declare no conflict of interest. (thermal reduction to produce oxygen). Freely available online through the PNAS open access option. The objective of our work is to create thermochemical water 1To whom correspondence should be addressed. E-mail: [email protected]. splitting cycles that involve non-corrosive solids and operate at This article contains supporting information online at www.pnas.org/lookup/suppl/ below 1,000 °C. Essentially, we wish to create new cycles that take doi:10.1073/pnas.1206407109/-/DCSupplemental. 9260–9264 ∣ PNAS ∣ June 12, 2012 ∣ vol. 109 ∣ no. 24 www.pnas.org/cgi/doi/10.1073/pnas.1206407109 aqueous solutions in the presence of bubbling CO2 at 80 °C; and (iv) recovery of Mn3O4 by thermally reducing the sodium ion extracted solid produced in step (iii) at 850 °C. The net reaction is the stoichiometric splitting of water to hydrogen and oxygen without any by-product. The incorporation and extraction of Naþ into and out of the manganese oxides are the critical steps in lowering the temperature required for both the hydrogen evo- lution and the thermal reduction steps (vide infra). Steps 1 and 2: Hydrogen evolution on Mn3O4∕Na2CO3. The presence þ 2þ 3þ of Na enables the oxidation of Mn in Mn3O4 to Mn by water, leading to the formation of α-NaMnO2,CO2, and H2.In the absence of Na2CO3, oxidation of Mn3O4 to Mn2O3 is always thermodynamically unfavorable (ΔG>0)(Fig.3A, top dotted line) (15). The introduction of Na2CO3 drastically changes the thermodynamics of the oxidation reaction; the ΔG of reaction Fig. 1. Isenthalpic and isentropic lines relating the temperature of the decreases with increasing temperature and becomes negative hydrogen generating step and the thermal reduction step. around 250 °C (Fig. 3A, solid line). These thermodynamic esti- mates are consistent with our experimental observations in that multistep, low-temperature water splitting cycle that has a highest water does not react with Mn3O4 in the absence of Na2CO3 at operating temperature of 850 °C. The thermochemical cycle 850 °C, and the amount of D2 obtained by reacting D2O(D2Ois consists of four main steps (Fig. 2): (i) thermal treatment of a used instead of H2O to enhance the signal-to-noise ratio in the product detection and quantification) with the Mn3O4∕Na2CO3 physical mixture of Na2CO3 and Mn3O4 to produce MnO, mixture at 850 °C is equivalent to the amount that would be CO2, and α-NaMnO2 at 850 °C; (ii) oxidation of MnO in the pre- 2þ 3þ expected when Mn is totally converted into Mn (Fig. 3B). sence of Na2CO3 by water to produce H2,CO2, and α-NaMnO2 3þ We hypothesize that Na2CO3 extracts the Mn from Mn3O4 iii þ α at 850 °C; ( )Na extraction from -NaMnO2 by suspension in at 500–850 °C to form α-NaMnO2,CO2, and MnO [Fig. 2, step 1; CHEMISTRY Fig. 2. Schematic representation of the low-temperature, Mn-based thermochemical cycle. Xu et al. PNAS ∣ June 12, 2012 ∣ vol. 109 ∣ no. 24 ∣ 9261 Fig. 4. Powder X-ray diffraction patterns used to identify solids phases in hydrogen evolution steps. (i) Solid collected after the hydrogen evolution step (after step 2); (ii) solid collected after reacting Mn3O4 with Na2CO3 at 850 °C (after step 1); (iii) sample from (ii) hydrolyzed in an aqueous suspen- sion in the presence of CO2 at 80 °C for 3 h; and (iv) sample from (iii) annealed at 180 °C in Ar for 1 h.
Details
-
File Typepdf
-
Upload Time-
-
Content LanguagesEnglish
-
Upload UserAnonymous/Not logged-in
-
File Pages15 Page
-
File Size-