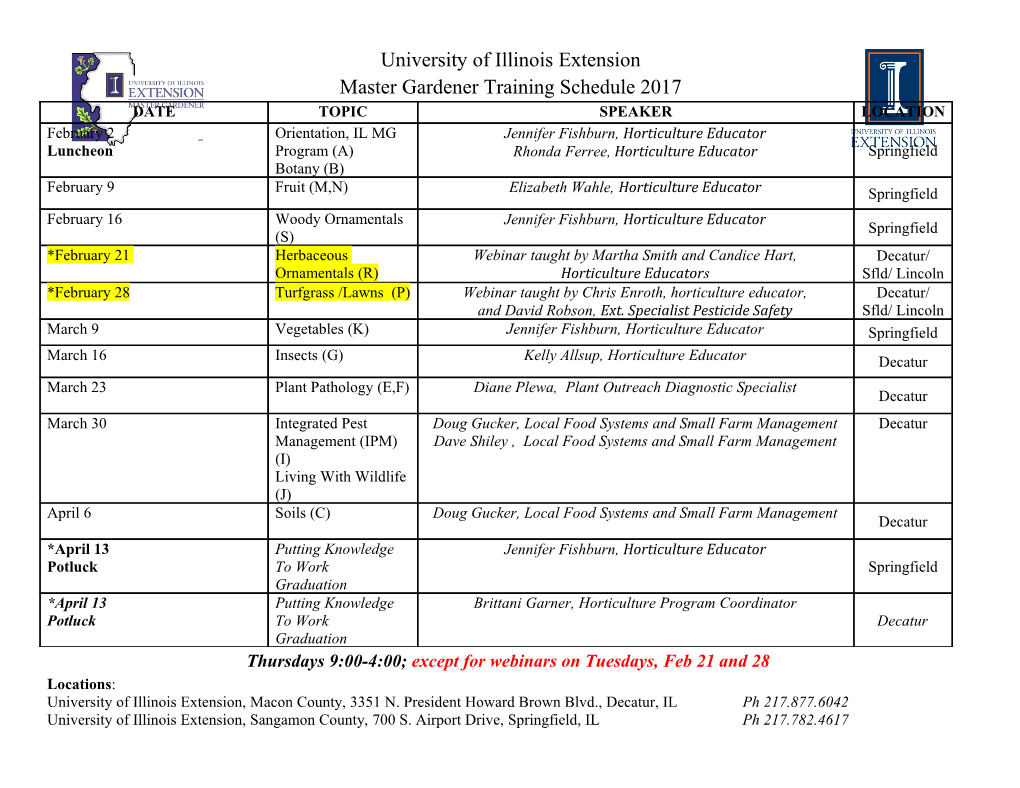
Introductory Lectures: Resurgence in Differential Equations, and Effective Summation Methods Gerald Dunne University of Connecticut Isaac Newton Institute Spring School: Asymptotic Methods and Applications, March 22-26, 2021 Isaac Newton Institute Programme: Applicable Resurgent Asymptotics, 2021/2022 Basic Introduction to Resurgence: A Beginner’s Guide Lecture 3 Basic Introduction to Resurgence: A Beginner’s Guide 1. Lecture 1: Resurgence & Linear Differential Equations I Trans-series and Stokes Phenomenon in ODEs I Borel Summation basics I Recovering Non-perturbative Connection Formulas 2. Lecture 2: Resurgence & Nonlinear Differential Equations I Nonlinear Stokes Phenomenon I Painlevé Equation examples I Parametric Resurgence & Phase Transitions 3. Lecture 3: Effective Summation Methods I Probing the Borel Plane Numerically I The Physics of Padé Approximation I Optimal Summation and Extrapolation Effective Summation Methods: A Basic Introduction • computing perturbative coefficients is difficult • how do we make practical use of resurgence in a really difficult problem where we can only compute a small number of perturbative coefficients ? • consider a series which appears to be asymptotic, with generic leading large order behavior of the coefficients Γ(a n + b) c ∼ S ; n ! 1 n An • A ! location of the leading Borel singularity • b ! nature of the leading Borel singularity • a ! appropriate expansion variable • the Stokes constant S! normalization Effective Summation Methods: A Basic Introduction PN cn • given just N terms of such a series n xn+1 (i) how can we sum accurately ? (ii) how can we extrapolate accurately to x = 0 ? (iii) how can we extrapolate into the complex x plane to probe Stokes transitions ? (iv) how can we extract non-perturbative exponentially small effects (connection) ? • resurgence suggests that local analysis of perturbation theory encodes global information • How much global information can be decoded from a FINITE number of perturbative coefficients ? • resurgent functions have orderly structure in Borel plane ) develop extrapolation and summation methods that take advantage of this Effective Summation Methods: A Basic Introduction • conclusion: it can make a BIG difference how we sum • processing the same perturbative input data in different ways can lead to vastly different levels of precision • the basic toolkit: ratio tests, series acceleration methods (e.g. Richardson), Padé approximants, orthogonal polynomials, Szegö asymptotics, continued fractions, conformal maps, uniformization maps, ... • the good news: many of these are actually very easy to implement ! a simple set of exploratory procedures • the quality of the extrapolation of an asymptotic series is governed by the quality of the analytic continuation of the Borel transform Z 1 f(x) = dt e−x tB[f](t) 0 • lesson 1: it is better to work in the Borel plane Resurgence in Nonlinear Differential Equations • empirical observation: for “natural problems” the Borel plane often has structure Padé-Borel • simple & powerful summation/extrapolation method 2N Z 1 2N X cn X cn = dt e−xt tn xn+1 n! n=0 0 n=0 Z 1 −xt = dt e B2N [f](t) 0 • recall that the singularities of B2N [f](t) determine the non-perturbative physics • but B2N [f](t) is a polynomial ! • as N ! 1, B2N [f](t) develops singularities • Padé is an excellent “low resolution” detector of singularity structures: “Padé-Borel” method Basics of Padé Approximation • simple and efficient method to analytically continue a series beyond its radius of convergence P2N n • rational approximation to function F2N (t) = n cn t 2N RL(t) X P fF g (t) = = c tn + O t2N+1 [L;M] 2N S (t) n M n • completely algorithmic and algebraic (“built-in”) • near-diagonal Padé: polynomials RN (t) & SN (t) satisfy the same 3-term recursion relation • hence a deep connection to orthogonal polynomials, and their asymptotics (Szegö ...) Basics of Padé Approximation 1. at very high orders Padé can be numerically unstable: a ratio of polynomials with very large coefficients. It is often more stable to convert to an ‘equivalent’ partial fraction 2N PN n N X n n an t X rn cnt $ $ PN n t − t n n dn t n n 2. truncated series ! continued fraction, which often converges in all of C, minus a number of poles/cuts 2N 2N+1 1 2N+1 1+c1 t+···+c2N t +O t = +O t h1 t 1 + h2 t 1+ h t 1+ 3 .. 1 .+h2N t 3. near-diagonal Padé are related to continued fractions Basics of Padé Approximation • a Padé approximant only has pole singularities. But for practical applications we are often interested in branch point singularities, e.g. Borel plane, critical exponents, ... • Padé represents a branch point as the accumulation point of an arc of poles: cf. potential theory (electrostatics) Im[t] Im[t] 10 10 5 5 Re[t] Re[t] -10 -5 5 10 -10 -5 5 10 -5 -5 -10 -10 1 1 1 1 Figure: Padé poles of 1 , 2πi 1 , and of 1 , 2πi 1 . (1−t) 5 (e 5 −t) 7 (1−t2) 5 (e 5 −t2) 7 Basics of Padé Approximation • symmetry of singularities is important for Padé Im[t] 2 1 Re[t] 5 10 15 -1 -2 Figure: Padé poles of 1=(1 − t + t2)1=5 • but the function has no singularities on the real t axis ! • Stahl (1997): in the limit N ! 1 Padé produces the “minimal capacitor” Im[t] 1.0 0.5 0.0 Re[t] 0.2 0.4 0.6 0.8 1.0 -0.5 -1.0 Figure: 1=t of Padé poles of 1=(1 − t + t2)1=5 Basics of Padé Approximation 1 • amazing sensitivity to exponent 2 1 1 inverse Padé poles of & 1 1 −10−6 (1 − t + t2) 2 (1 − t + t2) 2 Im[t] 1.0 0.5 0.0 Re[t] 0.2 0.4 0.6 0.8 1.0 -0.5 -1.0 • this sensitivity can be used to our advantage ... see later Basics of Padé Approximation Exercise 3.1: Explore the Padé pole structures for various functions with interesting singularities. Basics of Padé Approximation • Padé approximants are not very accurate near a branch point and branch cut, because of accumulating poles • Padé obscures repeated repeated singularities along the same line; but this is exactly what happens for resurgent Borel transforms in nonlinear problems • conformal mapping, before Padé, helps resolve these problems • make a conformal map into the unit disk, based on the leading Borel singularity/ies Conformal Mapping • conformal map of cut domains: t ! z Im[t] Im[t] Re[t] Re[t] Im[t] Im[z] Re[t] Re[z] Padé-Conformal-Borel Method • conformally map the Borel plane into the unit disk (based on the leading singularity), and then re-expand and do Padé inside the unit disk ) significant improvement for resurgent Borel transforms • precision can be quantified (Szegö asymptotics) • much better near singularities: higher precision determination of Stokes constants and resolution of the location and nature of the singularity • better extrapolation from x = +1 to x = 0 Basics of Padé Approximation 1 5 • cut of the Airy Borel function 2F1 6 ; 6 ; 1; −t • after the conformal map: Padé-Conformal-Borel: 10-term approximation to (1 + p)−1=3 Padé-Conformal-Borel: 10-term approximation to (1 + p)−1=3 • precision of the Borel transform along the cut Re[B(p)] 4 3 2 1 p -10 -8 -6 -4 -2 -1 -2 -3 blue: exact and Padé-Conformal-Borel approx. (10 terms) red: Padé-Borel approx. (10 terms) black-dashed: Taylor-Conformal-Borel approx. (10 terms) • Padé-Conformal-Borel is generically much more accurate near the singularity and along the cut Padé-Conformal-Borel: 10-term approx. in physical x plane • this translates into much better extrapolation of the Borel transform in the original physical x variable, from an expansion near x = +1 down to x ≈ 0 error 10 0.01 10-5 x 0.1 0.2 0.3 0.4 0.5 purple: 10 term x-Padé approx. (log of fractional error) red: 10 term Padé-Borel approx. blue: 10 term Padé-Conformal-Borel approx. black dotted line: 1% fractional error Padé-Conformal-Borel • the improved precision can be quantified −(Nx )1=2 cn ∼ Γ(n + α) ) frac. error ∼ e x-Padé 2 1=3 frac. error ∼ e−(N x) Padé-Borel 2 1=3 frac. error ∼ e−(N x) Taylor-Conformal-Borel 4 1=5 frac. error ∼ e−(N x) Padé-Conformal-Borel • for a chosen precision (e.g., 1% accuracy), with exactly the same input data we can extrapolate from an N-term expansion + at x = +1 down to xmin ! 0 , scaling with N as: extrapolation xmin scaling truncated series xmin ∼ N −1 x Padé xmin ∼ N −2 Padé-Borel xmin ∼ N −2 Taylor-Conformal-Borel xmin ∼ N −4 Padé-Conformal-Borel xmin ∼ N Padé-Conformal-Borel • another advantage of the conformal mapping is that it resolves repeated resurgent Borel singularities • recall that in a nonlinear problem a Borel singularity is expected to be repeated in (certain) integer multiples • these can be obscured by Padé’s attempt to represent a cut as an accumulation of poles Conformal Mapping of Borel Plane • map the doubly-cut Borel p plane to the unit disc p 2 z z = ! p = 1 + p1 + p2 1 − z2 Borel plane singularities: Padé-Borel transform Im[p] 10 5 Re[p] -0.4 -0.2 0.2 0.4 -5 -10 • before conformal map: resurgence is hidden After Conformal Map: Resurgent Poles in z Plane Im[z] 1.5 1.0 0.5 Re[z] -1.5 -1.0 -0.5 0.5 1.0 1.5 -0.5 -1.0 -1.5 • Conformal map reveals resurgent structure in Borel plane: Borel singularities separated into their resurgent patterns Optimal Method: Uniformizing Map • an even better procedure: replace the conformal map with a uniformizing map • Optimality Theorem (O.
Details
-
File Typepdf
-
Upload Time-
-
Content LanguagesEnglish
-
Upload UserAnonymous/Not logged-in
-
File Pages47 Page
-
File Size-