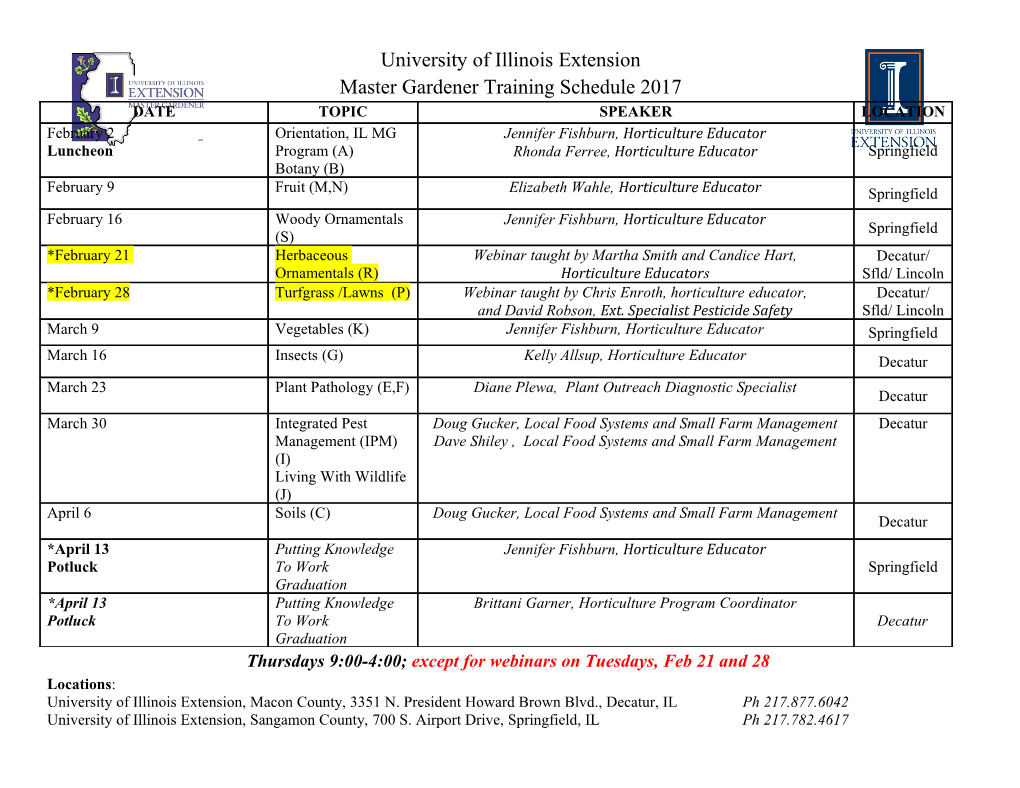
CHAPTER 12 CFD-based modeling of combustion and suppression in compartment fi res A. Trouvé & A. Marshall Department of Fire Protection Engineering, University of Maryland, College Park, USA. Abstract This chapter is aimed at illustrating contemporary computational fl uid dynamics (CFD) capabili- ties for compartment fi re applications. We choose to use the fi re dynamics simulator (FDS) for illustration purposes. FDS is developed by the National Institute of Standards and Technology, USA, and is one of the leading fi re simulation software available to fi re protection engineers and scientists; it is both representative of current capabilities as well as indicative of future trends. The material in this chapter is organized around a presentation of the different stages of a typi- cal enclosure fi re, from localized ignition to its fully developed state. We present simulations of model problems that serve to illustrate the performance and limitations of CFD-based descriptions for: (1) transient ignition phenomena and early fi re growth; (2) smoke fi lling and pre-fl ashover fi re spread; (3) possible fl ashover and transition to under-ventilated combustion; (4) activation of water-based fi re suppression systems and subsequent fi re control and/or extinction. 1 Introduction Fire safety is one of the engineering design problems considered during the construction and/or refurbishing of houses or buildings. Fires occurring in confi ned spaces exhibit unique features associated with smoke accumulation, restricted air ventilation, interactions with solid walls, and in many cases interactions with automatic fi re suppression systems. In the scientifi c literature, these wall-confi ned fi res belong to a special class of problems usually referred to as compartment or enclosure fi res. A typical compartment fi re scenario involves the following successive stages [1−3]: (1) ignition and early growth; (2) pre-fl ashover growth featuring a well-ventilated (i.e. fuel-limited) fi re and a hot smoke layer that develops near the compartment ceiling; (3) fl ashover that corresponds to a dramatic increase in the amount of burning liquid/solid materials; (4) post-fl ashover, fully devel- oped fi re dynamics featuring a ventilation-controlled (i.e. oxygen-limited) fi re. We briefl y review below the main physical features observed during the different stages of compartment fi res. WIT Transactions on State of the Art in Science and Engineering, Vol 31, © 2008 WIT Press www.witpress.com, ISSN 1755-8336 (on-line) doi:10.2495/978-1-84564-160-3/12 442 Transport Phenomena in Fires Stage 1 is diffi cult to describe in general terms because fi re problems feature a wide variety of possible ignition scenarios. The list includes scenarios dominated by heterogeneous processes (for instance, smoldering combustion as may occur in porous solid fl ammable materials) in which the incipient gas-phase combustion phenomena are strongly coupled with the thermally driven physical and chemical changes taking place within the liquid/solid fuel sources. It also includes scenarios dominated by gas-phase processes (for instance, processes occurring during ignition of fuel vapor clouds) in which transient combustion events correspond to a wide range of fl ame regimes, including premixed, non-premixed, and partially premixed combustion (PPC) modes. After ignition has occurred and a fi re is established (stage 2), fl ow confi nement and buoyancy forces lead to a natural vertical stratifi cation of the fi re room environment into two layers: a ceil- ing layer resulting from the accumulation of high temperature combustion products mixed with entrained air; and a fl oor layer corresponding to fresh air at ambient (or slightly preheated) tem- perature. The high temperatures found in the ceiling layer contribute to the intensifi cation of the heat feedback experienced by fl ammable objects/materials present in the fi re room, and thereby to a faster fi re growth. Fire growth is gradual at fi rst, until critical conditions are reached, at which point it becomes very rapid. This rapid increase in fi re size is called fl ashover (stage 3); fl ashover may be interpreted as a series of spontaneous ignition events driven by super-critical levels of irradiation from super-hot ceiling layer gases (i.e. gases with temperatures in excess of 800−900 K). After fl ashover, virtually all fl ammable objects and materials present in the room are involved in the fi re. The amount of gaseous fuel mass generated by the thermal degradation processes occurring in liquid or solid fl ammable sources (i.e. as a result of evaporation or pyrolysis pro- cesses) may then be so large that the combustion dynamics become fuel rich, i.e. oxygen-limited (stage 4). Under oxygen-limited fi re conditions, the fl ame may experience a dramatic change and migrate from the fuel sources to the compartment vents location; this transition is similar to the fl ame opening process observed in Burke−Schumann type laminar diffusion fl ames when going from fuel-lean to fuel-rich conditions. In addition, in typical under-ventilated fi re situations, large sections of the fl ame are supplied with vitiated air, i.e. with a mixture of pure air and re-circulating combustion products. Suffi cient levels of air vitiation will result in sub-critical oxygen concentra- tions and consequent partial or total fl ame extinction. Air vitiation effects stress the importance of oxygen depletion for the fl ame dynamics, and explain why a fl ame that develops in a sealed or poorly ventilated space will ultimately experience quenching. Finally, during stage 4, since only a fraction of the fuel mass generated in the fi re room actually burns there, the excess (unburnt) fuel mass may be transported into adjacent rooms through vents and openings. This leakage of fuel mass may in turn lead to burning outside the room of fi re origin, and thereby contribute to fi re spread to adjacent spaces. In this chapter we examine the ability of current computational fl uid dynamics (CFD) approaches to simulate the different physical features observed in compartment fi res. We choose to use the fi re dynamics simulator (FDS) for illustration purposes. FDS is developed by the Building and Fire Research Laboratory (BFRL) of the National Institute of Standards and Tech- nology (NIST), USA; it is available as freeware [4] and is oriented towards fi re applications [5−7]. It is worth emphasizing that our selection of FDS is not meant as an endorsement of FDS over other CFD tools also available for simulations of building fi res (see refs [8, 9] for examples of results obtained using other CFD tools). FDS is adopted in this study because of its popularity and availability, and because it is representative of current fi re modeling capabilities. The main features of FDS include a large eddy simulation (LES) approach to treat turbulent fl ow motions (based on the turbulent eddy diffusivity concept and the classical Smagorinsky WIT Transactions on State of the Art in Science and Engineering, Vol 31, © 2008 WIT Press www.witpress.com, ISSN 1755-8336 (on-line) CFD-Based Modeling of Combustion and Suppression 443 closure model); a low Mach number formulation to handle fl ow compressibility (and to fi lter out fast moving and computationally demanding acoustic waves); a second-order fi nite difference scheme for spatial discretization; a second-order predictor−corrector explicit scheme for time integration; a multi-block, rectangular Cartesian grid capability; a parallel computing capability based on the message passing interface (MPI) protocols. The offi cial release of FDS includes a mixture-fraction-based model proposed to describe non-premixed turbulent combustion; this model includes a fl ame extinction capability due to air vitiation [5, 6]. In this study, we also used a modifi ed in-house version of FDS that has enhanced modeling capabilities and can treat multi- mode (i.e. partially premixed) combustion. All computational results presented herein were gen- erated by running FDS in parallel mode using fi ve processors on a Linux PC cluster; the cluster is equipped with 2.4−3.8 GHz Intel Xeon processors and was made available to us by BFRL/ NIST. The material in this chapter is organized around a presentation of the different stages of a typical enclosure fi re, from localized ignition to its fully developed state. We present simulations of model problems that serve to illustrate the current performance and limitations of CFD-based descriptions for: transient ignition phenomena and early fi re growth (Section 2); smoke fi lling and pre-fl ashover fi re spread (Section 3); possible fl ashover and transition to under-ventilated combustion (Section 4); activation of water-based fi re suppression systems and subsequent fi re control and/or extinction (Section 5). 2 Transient ignition and early fi re growth In this section, we examine the feasibility of a CFD approach to simulate transient ignition events, as might occur in fi re and explosion safety scenarios. Note that out of the long list of possible igni- tion scenarios, we limit the scope of our discussion hereafter to gas-phase processes. We consider a case corresponding to the hazardous accumulation of fuel vapor in a room (Fig. 1); this accumu- lation may be the result of an accidental liquid fuel spill, assuming that the liquid fuel has a low fl ashpoint temperature and undergoes spontaneous evaporation under room ambient conditions. We choose to assume that the room is closed, although many of the following developments would also apply to scenarios occurring in vented rooms or unconfi ned spaces. We also assume in the following that: there is a signifi cant delay between the start of the fuel build-up and the ignition event, thereby allowing the formation of a sizeable fuel vapor cloud prior to combustion; the fuel- air mixing process results in some large portion of the fuel vapor cloud being within the fuel-air fl ammability limits; ignition occurs at some location in the fl ammable portion of the fuel vapor cloud.
Details
-
File Typepdf
-
Upload Time-
-
Content LanguagesEnglish
-
Upload UserAnonymous/Not logged-in
-
File Pages37 Page
-
File Size-