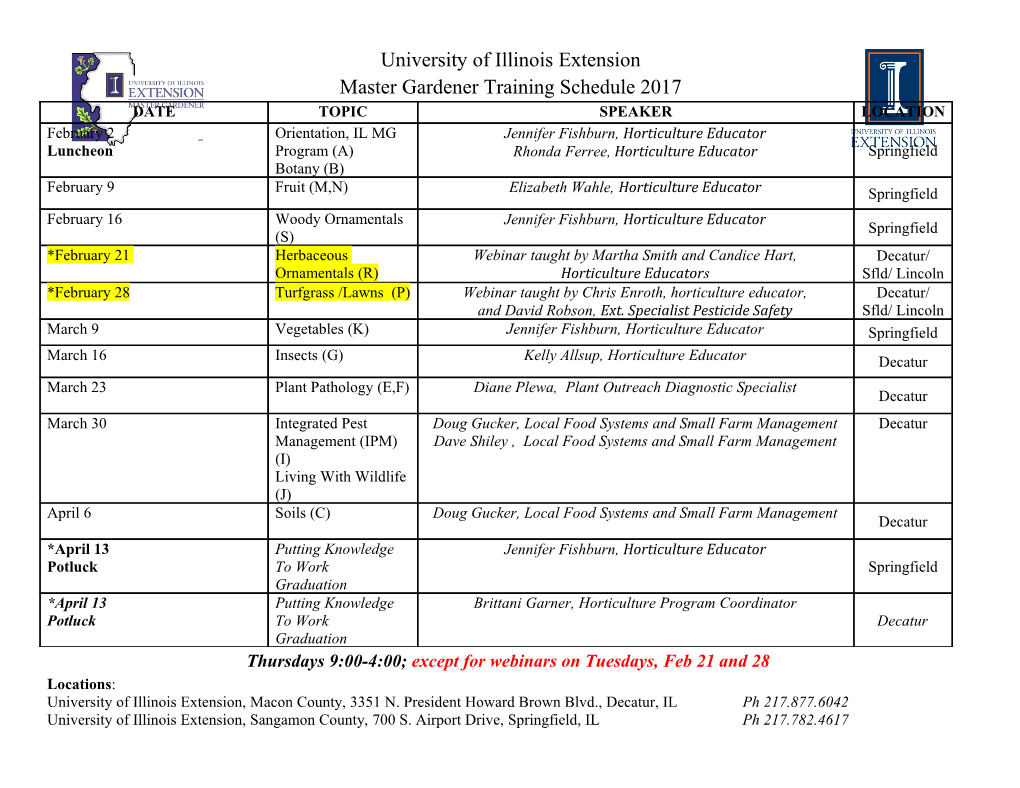
Journal of Glaciology Meltwater percolation, impermeable layer formation and runoff buffering on Devon Ice Cap, Canada Paper David W. Ashmore1, Douglas W. F. Mair1 and David O. Burgess2 Cite this article: Ashmore DW, Mair DWF, 1School of Environmental Sciences, Roxby Building, University of Liverpool, Liverpool L69 7ZT, UK and 2Natural Burgess DO (2020). Meltwater percolation, Resources Canada, 601 Booth St., Ottawa, Ontario K1A 0E8, Canada impermeable layer formation and runoff buffering on Devon Ice Cap, Canada. Journal of Glaciology 66(255), 61–73. https://doi.org/ Abstract 10.1017/jog.2019.80 The retention of meltwater in the accumulation area of the Greenland ice sheet and other Arctic Received: 13 March 2019 ice masses buffers their contribution to sea level change. However, sustained warming also results Revised: 11 October 2019 in impermeable ice layers or ‘ice slabs’ that seal the underlying pore space. Here, we use a 1-D, Accepted: 14 October 2019 physically based, high-resolution model to simulate the surface mass balance (SMB), percolation, First published online: 8 November 2019 refreezing, ice layer formation and runoff from across the high-elevation area of Devon Ice Cap, Key words: Canada, from 2001 to 2016. We vary the thickness of the ‘impermeable’ ice layer at which Arctic glaciology; melt – surface; polar firn; underlying firn becomes inaccessible to meltwater. Thick near-surface ice layers are established snow/ice surface processes; surface mass by an initial deep percolation, the formation of decimetre ice layers and the infilling of interleav- budget ing pore space. The cumulative SMB increases by 48% by varying impermeable layer thickness – Author for correspondence: between 0.01 and 5 m. Within this range we identify narrower range (0.25 1 m) that can simulate David W. Ashmore, both the temporal variability in SMB and the observed near-surface density structure. Across this E-mail: [email protected] range, cumulative SMB variation is limited to 6% and 45–49% of mass retention takes place within the annually replenished snowpack. Our results indicate cooler summers after intense mid-2000s warming have led to a partial replenishment of pore space. Introduction The Arctic is warming at twice the global mean leading to widespread ice mass loss, largely attributable to increased surface melt (e.g. van den Broeke and others, 2016). However, not all surface meltwater becomes proglacial runoff. At higher elevations, in the accumulation zone, meltwater can percolate into porous snow and firn. Meltwater may be retained as liquid water, but is more typically retained as infiltration ice, i.e. water that has percolated through the porous snowpack to refreeze as distinct high-density ice lenses or layers. Meltwater in the accumulation area that does not refreeze percolates to an impermeable horizon and migrates laterally, eventually entering the glacier hydrological system. The proportioning of melt to that which runs off or is retained is often considered a function of ice ‘cold content’ and pore space (e.g. van Angelen and others, 2013). However, the effect of existing density stratigraphy and grain properties on controlling retention is less well-known (e.g. Bell and others, 2008, Machguth and others, 2016). In the warming Arctic, areas of previously dry snow are now routinely experiencing melt and so elucidating processes controlling meltwater percolation, refreezing and runoff in an evolving stratigraphy is critical for improving projec- tions of sea level rise. Recent estimates suggest that the top 10 m of the Greenland ice sheet may store ∼6500 km3 of meltwater and buffer its future contribution to sea level (Vandecrux and others, 2019). Projections using a regional climate model coupled with a firn model indicate that by the end of 21st century meltwater will fill 50% of Greenland’s pore space, reducing the near- surface refreezing capacity and accelerating mass loss (van Angelen and others, 2013). Field evidence has revealed a densification of firn zones (e.g. de la Peña and others, 2015) and that some meltwater may percolate past or through ice layers several decimetres thick (Humphrey and others, 2012). However, layers of ice several metres thick (also known as ice slabs) may form and seal pore space from most subsequent percolating meltwater (Gascon and others, 2013a; Machguth and others, 2016; MacFerrin and others, 2019). It is unclear, therefore, what constitutes a barrier to percolation in the accumulation zone. Field measurements are difficult to interpret in studies of accumulation area stratigraphy due to extreme spatial and temporal heterogeneity in the processes of meltwater percolation and © The Author(s) 2019. This is an Open Access refreezing (Scott and others, 2006; Bell, 2008; Bell and others, 2008; Brown and others, article, distributed under the terms of the Creative Commons Attribution licence (http:// 2011). From temporally discontinuous firn core and snow pit data it is not possible to identify creativecommons.org/licenses/by/4.0/), which whether an ice layer has grown from freezing-on from the top, or by percolation through and permits unrestricted re-use, distribution, and freezing-on from below a pre-existing ice layer. Snow and firn evolution models have value reproduction in any medium, provided the here as they allow the continuous investigation of the development of near-surface ice layers, original work is properly cited. which can be used to place sporadic and hard-won field measurements in context. In this paper we compare results from a calibrated surface energy and mass-balance model to field data to explore the sensitivity of the surface mass balance (SMB) of Devon Ice Cap (DIC), cambridge.org/jog Canada to the dynamic, physical stratigraphy of its near-surface snow and firn. Downloaded from https://www.cambridge.org/core. 30 Sep 2021 at 06:31:19, subject to the Cambridge Core terms of use. 62 David W. Ashmore and others Approaches to modelling snow and firn evolution Study area The application of a simple distributed model to understand the The Canadian Arctic Archipelago contains the largest reservoir of firn dynamics of a remote ice cap necessarily involves several land ice outside the great ice sheets and currently contributes − assumptions and omissions. Many firn models (e.g. Bougamont ∼60 Gt a 1 to global sea level rise (Harig and Simons, 2016). and others, 2005; Ettema and others, 2010) including ours, are Many glaciers and ice caps have been losing mass since ∼1960s based on Greuell and Konzelmann (1994) and the ‘tipping-bucket’ in the Canadian Arctic (Koerner, 2005; Mair and others, 2005, scheme. Here, melt instantaneously percolates in porous snow- 2009; Noël and others, 2018). However, since 2005 mass loss packs, sometimes after an irreducible water content is exceeded, from these glaciers and ice caps has accelerated (Gardner and to refreeze when there is sufficient ‘cold content’. With this, we others, 2011; Sharp and others, 2011) and has undergone intense place our modelling efforts presented in this paper in context melt at levels likely unseen for several millennia (Fisher and with other recent approaches. Avalanche and seasonal snowpack others, 2012). Ground penetrating radar (GPR) and firn core research has typically prioritised accuracy at specific sites utilising measurements have shown that surface ice layers several metres a range of physical parameterisations (e.g. Wever and others, 2014). thick have formed within DIC in the last decade (Bezeau and One focus has been on the role of preferential flow paths and others, 2013; Gascon and others, 2013a). This clearly marks their inclusion in model development, motivated in part by the DIC as a target for further investigations of snow and firn observation of apparent refreezing events at several metres depth dynamics in the accumulation area. in instrumented boreholes drilled through firn containing ice DIC is situated on the eastern edge of Devon Island, Nunavut layers several decimetres thick (e.g. Humphrey and others, 2012). in the Canadian Arctic Archipelago (Fig. 1). It is 13 700 km2 in The development of preferential flowpaths is likely related to the area as delineated by 2014 Landsat-8 imagery, and has a max- effect of snow structure and capillary barriers and so is difficult imum elevation of 1930 m. The ice cap has both land and marine to apply in data poor regions in a physically based manner margins and a single east-west trending major ice divide. DIC (Waldner and others, 2004; Katushima and others, 2013; Avanzi experiences cold winters with low accumulation (∼100 kg m2) and others, 2016). One strand of research has sought to treat matrix resulting in surface processes during summer dominating its flow and preferential flow as two model domains with exchange mass balance (Koerner, 2005). Since the mid-2000s summer between them (Wever and others, 2016; Marchenko and others, melt has accelerated (Gascon and others, 2013b) and increased 2017). A different approach is discussed by Meyer and Hewitt DIC mass loss (Gardner and Sharp, 2009). Intense surface melt (2017) who present a continuum model contrasting to the com- and percolation has led to the development of layers of massive mon cell-based approaches. In this model pore space is filled ice several metres thick in the near-surface. Sylvestre and others according to a Darcian permeability and presupposes that runoff (2013) also attribute ice layer growth to a large rain event in occurs at the ice surface. The use of the Richards equations, origin- 2006. Repeat GPR surveys 2007–2012 suggest that after a deep ally derived to model the movement of water in partially saturated (5–6 m) percolation event, gaps between ice layers were initially soils, have recently been applied to a seasonal snowpack to predict filled with refrozen melt, before the ice layer subsequently thick- runoff and it is unclear how applicable they are to snow hydrology ened by vertical accretion (Gascon and others, 2013a).
Details
-
File Typepdf
-
Upload Time-
-
Content LanguagesEnglish
-
Upload UserAnonymous/Not logged-in
-
File Pages13 Page
-
File Size-