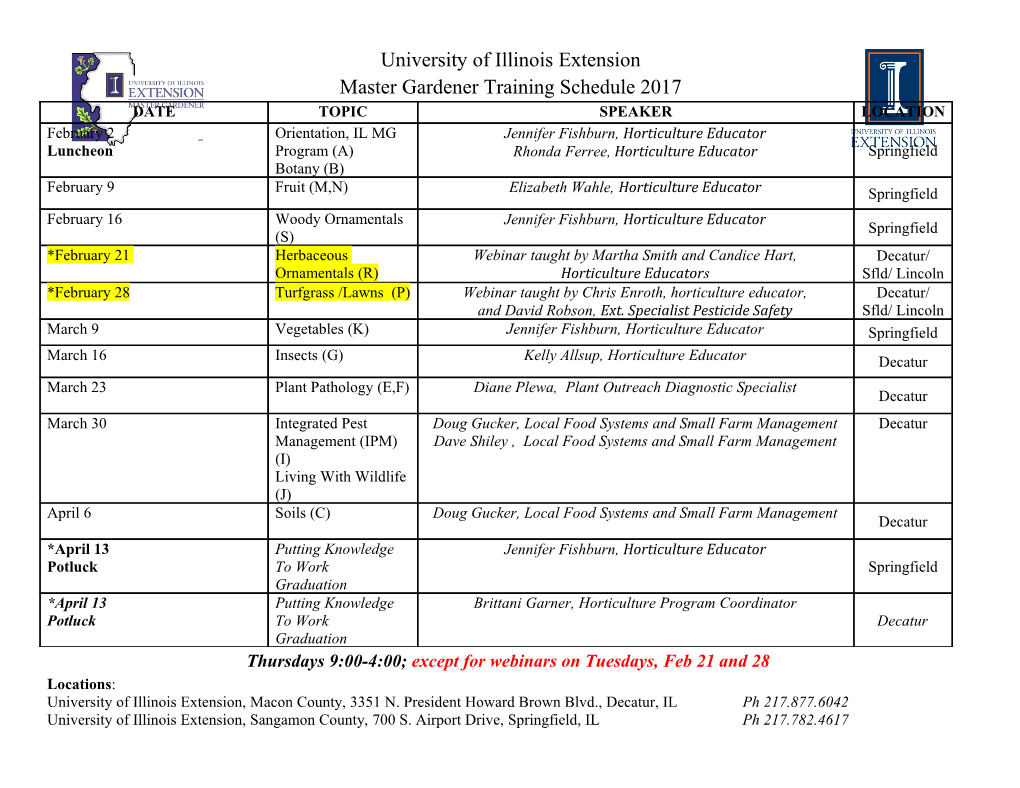
THE CONDUCTOR IDEAL KEITH CONRAD 1. Introduction Let O be an order in the number field K. When O 6= OK , O is Noetherian and one- dimensional, but is not integrally closed. The ring O has at least one nonzero prime ideal that's not invertible and some nonzero ideal in O does not have a unique prime ideal factorization, since otherwise O would be a Dedekind domain and thus would be integrally closed. We will define a special ideal in O, called its conductor, that is closely related to the noninvertible prime ideals in O. The nonzero ideals in O that are relatively prime to the conductor are invertible in O and have unique factorization into prime ideals in O. Definition 1.1. The conductor of an order O in the number field K is c = cO = fx 2 K : xOK ⊂ Og: This is a subset of O since 1 2 OK , so c = fx 2 OK : xOK ⊂ Og = fx 2 O : xOK ⊂ Og; and the last formula for c shows us that c is the annihilator of OK =O as an O-module: c = AnnO(OK =O): Example 1.2. Let K = Q(i) and O = Z[2i] = Z + Z2i. For x = a + 2bi in O, where a and b are integers, we have xZ[i] ⊂ O if and only if xi 2 O, which is equivalent to a being even. Therefore c = f2m + 2ni : m; n 2 Zg. 1 Writing the condition x 2 c as OK ⊂ x O when x 6= 0, the conductor is the set of all common denominators when we write the algebraic integers of K as ratios from O, together with 0. Since K is the fraction field of O and OK is a finitely generated O-module (because it is a finitely generated Z-module) there are such common denominators, so c 6= f0g. Explicitly, since the index m = [OK : O] is finite, mOK ⊂ O, so m 2 c. The conductor is an ideal in O. Since O ⊂ OK , we have 1 2 c if and only if O = OK . So c is a proper ideal in O when O is a nonmaximal order. The conductor of O is also an ideal in OK : if x 2 c and α 2 OK then αxOK ⊂ xOK ⊂ O, so αx 2 c. A nonzero ideal of one ring that is also an ideal of a larger ring might seem a bit peculiar. (The principal ideals (3) in Z[i] and Z[2i] are written the same way but are not the same; the first contains 3i and the second does not.) The property of c being an ideal in both O and OK leads to a characterization of it: Theorem 1.3. An ideal in OK lies in O if and only if it is contained in c, so c is the largest ideal of OK that is contained in O. Proof. If a is an ideal in OK that lies in O then aOK = a ⊂ O, so a ⊂ c. Conversely, an ideal in OK that is contained in c is contained in O since c ⊂ O. 1 2 KEITH CONRAD Remark 1.4. While every ideal of OK that lies in O is inside c, not all ideals of O that lie in c are ideals of OK . When O = Z[2i] = Z + Z2i, the ideal 2O = Z2 + Z4i of O is contained in c = Z2 + Z2i but 2O is not an ideal in Z[i] since it contains 2 but not 2i. 2. Examples Example 2.1. Let K be a number field, a be a nonzero ideal in OK and set O = Z + a, so a ⊂ O ⊂ OK . Letting a \ Z = aZ, we will show c = dZ + a where d is a certain factor of a. Since a ⊂ O we have a ⊂ c by Theorem 1.3. By the ring isomorphism O=a = (Z + a)=a =∼ Z=aZ, each ideal in O containing a is dZ + a where d divides a. Therefore c = dZ + a for some d dividing a. What is this d? Since a \ Z = aZ, d is the smallest positive integer in c. The condition d 2 c is the same as dOK ⊂ O, and such integers d are the multiples of the exponent of OK =O (the exponent of a finite abelian group is the least integer annihilating the whole group). So as d we can use the exponent of OK =O: O = Z + a =) cO = dZ + a; where d = exponent of OK =O: + As a special case, consider a = cOK with c 2 Z and take K 6= Q, so n = [K : Q] ≥ 2. We will show the order O = Z + cOK has conductor cOK . To determine the exponent of Ln OK =O, write OK = i=1 Zei, where e1 = 1 (there is always some Z-basis of OK containing Ln ∼ Ln ∼ n−1 1.) Then O = Z ⊕ i=2 Zcei, so OK =O = i=2 Z=cZ = (Z=cZ) , which as an abelian group has exponent c (since n ≥ 2), so O = Z + cOK =) cO = cZ + cOK = cOK : ∼ This conductor is a principal ideal in OK but it is not principal as an ideal in O: O=cOK = n n Z=cZ while each nonzero α 2 cOK has norm a multiple of c , so [O : αO] ≥ c > c. The index formula [Z + cOK : cOK ] = c tells us when c = p is prime that pOK is a prime ideal in Z + pOK . When K is a quadratic field, every order in K has the form Z + cOK for a unique c ≥ 1. The conductor of this order is cOK and c = [OK : Z + cOK ] (because [K : Q] = 2). It is standard to label the conductor as c rather than cOK . This is special to the quadratic case. That is, for orders in quadratic fields the label \conductor" and \index" (in OK ) mean the same thing. For example, the order of conductor 2 in Q(i) is Z[2i] and the order p p p 1+ 5 of conductor 2 in Q( 5) is Z[2 2 ] = Z[ 5]. Orders of the form Z + aOK with a 2 Z − f0g have conductors that are principal ideals in OK . To find a conductor that is not a nonprincipal ideal in OK , we look at cubic orders. p p 3 3 3 Example 2.2.p Let K = Q( 7). The ring of integers is OK = Z[ 7] and (3) = p , where p = (3; 1 − 3 7). Set O = Z + p2. Since p2 \ Z = 3Z, O=p2 =∼ Z=3Z, so [O : p2] = 3, which means p2 is a prime ideal in O. (It looks a bit strange to say p2 is a prime ideal, but we're in the ring O, where p isn't even 2 2 a subset.) Since [OK : p ] = N(p) = 9, [OK : O] = 3, a prime, so OK =O has exponent 3. 2 2 Byp Example 2.1, the conductor of O is c = 3Z +p p = p . This is not a principal ideal in Z[ 3 7] since it has norm 9 and no element of Z[ 3 7] has norm ±9: p p 3 3 3 3 3 NK=Q(a + b 7 + c 49) = a + 7b + 49c − 3 · 7abc; p p so if a + b 3 7 + c 3 49 has norm ±9 we reduce mod 7 to get a3 ≡ ±9 mod 7, which has no solution. THE CONDUCTOR IDEAL 3 p Example 2.3. Let K = Q( 3 19). The ring of integers is p p 2 p 1 + 3 19 + 3 19 (2.1) O = Z + Z 3 19 + Z : K 3 p We will compute the conductor c for the order Z[ 3 19]. This order has not been constructed as Z + a, so we can't appeal to Example 2.1 to compute c. Instead we will compute c using the definition of the conductor. For x = p p p p 3 3 2 3 3 a + b 19p + c 19 in Z[ 19], to have x 2 c means xOK ⊂ Z[ 19], which is equivalent to 3 xei 2 Z[ 19], where e1; e2; e3 is a Z-basis of OK . Using the visible Z-basis of OK in (2.1) leads to the conditions a; b; c, and (a + b + c)=3 2 Z. Writing a + b + c = 3d, p 2 p p 2 p 2 x = a(1 − 3 19 ) + b( 3 19 − 3 19 ) + d · 3 3 19 ; so p 2 p p 2 p 2 (2.2) c = Z(1 − 3 19 ) + Z( 3 19 − 3 19 ) + Z · 3 3 19 : Expressing the Z-spanning set for c in (2.2) in terms of the Z-basis of OK , p 0 3 2 1 0 1 0 1 1 − 19 2 1 −3 p1 p p 2 3 B 3 19 − 3 19 C = @ 1 2 −3 A @ 19 A : @ p A p p 2 3 3 19 0 3 0 (1 + 3 19 + 3 19 )=3 The matrix has determinant 9, so [OK : c] = 9: the ideal c in OK has norm 9. We show by contradiction that c is not principalp in OpK . If c = αOK for some α 2 c, 3 3 9 = [OK : αOK ] = j NK=Q(α)j. Writing α = a + b 19 + c 19 with integers a; b, and c, 3 3 2 3 NK=Q(α) = a + 19b + 19 c − 3 · 19abc; which reduces mod 19 to a3. Since ±9 mod 19 is not a cube, we have a contradiction.
Details
-
File Typepdf
-
Upload Time-
-
Content LanguagesEnglish
-
Upload UserAnonymous/Not logged-in
-
File Pages15 Page
-
File Size-