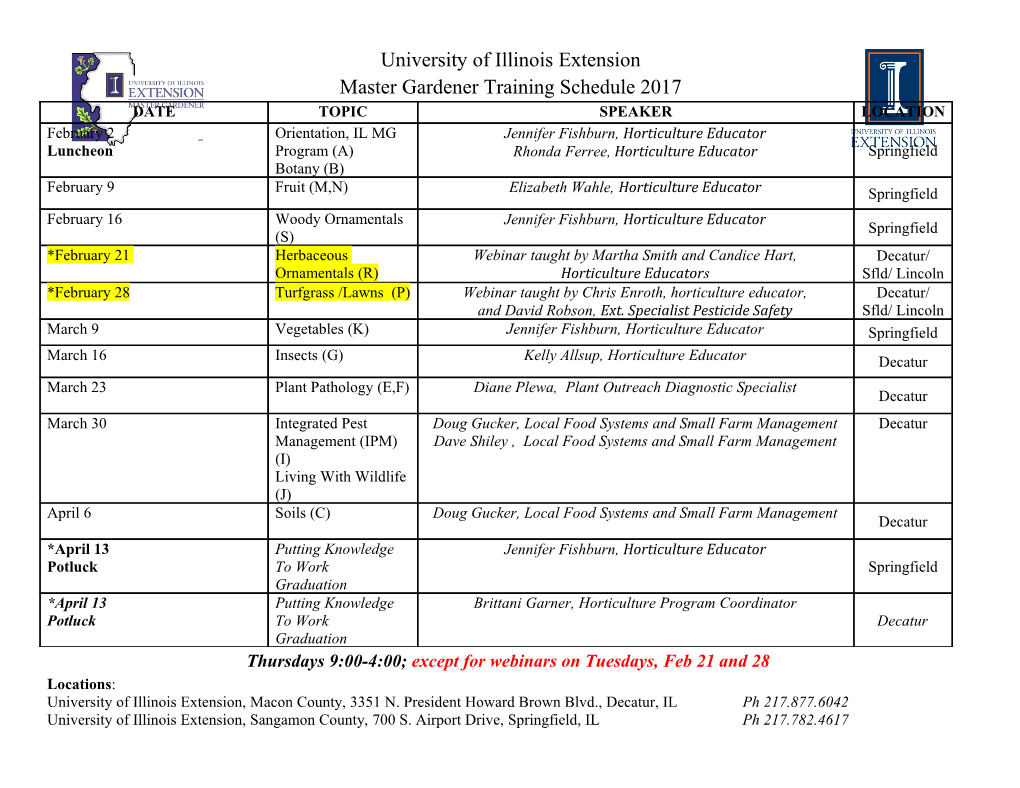
DNA energy landscapes via calorimetric detection of microstate ensembles of metastable macrostates and triplet repeat diseases Jens Vo¨ lkera, Horst H. Klumpb, and Kenneth J. Breslauera,c,1 aDepartment of Chemistry and Chemical Biology, Rutgers, The State University of New Jersey, 610 Taylor Rd, Piscataway, NJ 08854; bDepartment of Molecular and Cell Biology, University of Cape Town, Private Bag, Rondebosch 7800, South Africa; and cCancer Institute of New Jersey, New Brunswick, NJ 08901 Communicated by I. M. Gelfand, Rutgers, The State University of New Jersey, Piscataway, NJ, October 15, 2008 (received for review September 8, 2008) Biopolymers exhibit rough energy landscapes, thereby allowing biological role(s), if any, of kinetically stable (metastable) mi- biological processes to access a broad range of kinetic and ther- crostates that make up the time-averaged, native state ensembles modynamic states. In contrast to proteins, the energy landscapes of macroscopic nucleic acid states remains to be determined. of nucleic acids have been the subject of relatively few experimen- As part of an effort to address this deficiency, we report here tal investigations. In this study, we use calorimetric and spectro- experimental evidence for the presence of discrete microstates scopic observables to detect, resolve, and selectively enrich ener- in metastable triplet repeat bulge looped ⍀-DNAs of potential getically discrete ensembles of microstates within metastable DNA biological significance. The specific triplet repeat bulge looped structures. Our results are consistent with metastable, ‘‘native’’ ⍀-DNA species investigated mimic slipped DNA structures DNA states being composed of an ensemble of discrete and corresponding to intermediates in the processes that lead to kinetically stable microstates of differential stabilities, rather than DNA expansion in triplet repeat diseases (36). Incorrect pro- exclusively being a single, discrete thermodynamic species. This cessing by the cellular replication and repair machinery of such conceptual construct is important for understanding the linkage transiently formed slipped structures, in their macro and/or between biopolymer conformational/configurational space and microstate ensembles, is believed to cause DNA expansion, the biological function, such as in protein folding, allosteric control of genotype that ultimately leads to the phenotype associated with enzyme activity, RNA and DNA folding and function, DNA structure DNA expansion diseases (37–50). The presence of discrete and and biological regulation, etc. For the specific DNA sequences and kinetically stable microstates within the ensemble of the triplet structures studied here, the demonstration of discrete, kinetically repeat macrostate is therefore of potential significance for stable microstates potentially has biological consequences for understanding the biology of triplet repeat diseases. understanding the development and onset of DNA expansion and triplet repeat diseases. Results and Discussion We have shown that the 2 complementary (CAG)6 and (CTG)6 calorimetry ͉ nucleic acid conformations triplet repeat bulge looped ⍀-DNAs diagrammed in Fig. 1A do not spontaneously rearrange to their thermodynamically more he concept of rough biopolymer energy landscapes is im- stable duplex states when combined in solution, but rather Tportant for understanding biopolymer properties and func- remain trapped in their metastable looped structures (36). tion. Complex landscapes allow partial population of discrete, Strand exchange to the duplex state requires input of energy, kinetically stable (metastable) species (1–12). These substates, in occurring only at elevated temperatures. At the so-called ex- turn, may serve as biologically significant ligands, beyond the change temperature, Tex, the strand exchange reaction proceeds traditional, thermodynamically most stable, time-averaged, ‘‘na- irreversibly, with a release of heat energy. We detect this strand tive’’ macrostrate. Although it is well recognized that macro- exchange between the 2 metastable bulge looped ⍀-DNAs to scopic states are composed of microscopic substates, it has been form the 2 fully paired duplex states by monitoring the unusual customary for experimentalists to think of a biologically relevant exothermic peak in the DSC curves that, as expected for an native state as a singular macrostate with a unique, well defined irreversible process, is absent in the second heating scan structure characterized by a homogeneous/smooth energy pro- (Fig. 1B). file. Many biophysical measurements probe properties that are These observations are consistent with the energy landscape averaged over the ensemble of microstates that collectively of these looped, repeat sequences containing local minima (here correspond to a macroscopic native state. However, experimen- the ⍀-DNA structures), in addition to the global Watson and tal evidence for the presence of, and dynamic interchange Crick duplex minima. Such metastable states may well present between, multiple states that constitute a global macrostate is processing problems for the machinery of replication and repair. provided by direct time-resolved measurements, and by struc- Further heating of the system results in conventional duplex tural dispersions in crystallographic and NMR studies (13–22). melting, fully consistent with expectation. Such results underscore the potential importance as ligands or The landscape for these transformations is depicted in the receptors in biological mechanisms of kinetically stable, trapped energy funnel shown in Fig. 2. The arrow in this figure tracks the microstates embedded within the rough energy landscapes of transformations noted above; namely, from the ensemble of high purportedly singular macrostates. For example, the detection of energy single strands (corresponding to the upper outer rim of transiently populated structural states in the native unbound protein ensemble that resemble those of the proteins in their bound state provides evidence for the so-called ‘‘conformational Author contributions: J.V., H.H.K., and K.J.B. designed research; J.V. performed research; selection model’’ of protein binding, thereby suggesting signifi- J.V., H.H.K., and K.J.B. analyzed data; and J.V. and K.J.B. wrote the paper. cant biological functions for microstates in proteins (23–26). The authors declare no conflict of interest. By contrast with proteins, relatively few studies have probed Freely available online through the PNAS open access option. the energy landscapes of nucleic acids, particularly via calori- 1To whom correspondence should be addressed. E-mail: [email protected]. metric techniques (27–35). As a result, the potential energetic/ © 2008 by The National Academy of Sciences of the USA 18326–18330 ͉ PNAS ͉ November 25, 2008 ͉ vol. 105 ͉ no. 47 www.pnas.org͞cgi͞doi͞10.1073͞pnas.0810376105 Downloaded by guest on September 28, 2021 Fig. 2. Idealized representation of an energy landscape, showing features related to the triplet repeat DNA conformations investigated here (4). For clarity of presentation and ease of interpretation, the energy wells and the idealized trajectories between them are shown as smooth surfaces. The experimental data reported here, however, reveal that these surfaces are not smooth. The specific trajectory by which the DNA strands combine/fold and the shape of the folding landscape will depend on the temperature. At temperatures above exchange (landscape not shown), the barrier between the local ⍀-DNA minimum, and the global duplex minimum would be dimin- ished such that the ⍀-DNA state no longer would be populated. [Reproduced with permission from ref. 4 (Copyright 1998, Wiley, New York).] change between the 2 ⍀-DNAs is irreversible, any exotherm detected in the postincubation second scan reflects the unre- acted/unexchanged fractions of the original 2 ⍀-DNAs. The results obtained here for different incubation temperatures are shown in Figs. 3A and 3B and are described below. We find that when the sample is incubated at temperatures well below the onset of the exchange reaction, the ⍀-DNAs do not undergo strand exchange during the incubation period, as evi- denced by the presence of the full exothermic peak in the DSC rescan curve (the black curve in Fig. 3A). Conversely, when the Fig. 1. Interconversions of DNA states. (A) Schematic representation of the sample is incubated at temperatures above the exchange temper- different DNA conformations and the transformations between them. (B) ature, all of the ⍀-DNA molecules undergo strand exchange, as Calorimetric excess heat capacity curve for the first DSC heating scan of a 1:1 evidenced by no signal being observed for residual, unreacted ⍀ mixture of 2 complementary, triplet-repeat bulge looped -DNAs. The dotted ⍀-DNAs in the DSC rescan (the magenta curve in Fig. 3A). vertical lines delimit the thermal domains in which particular DNA conforma- tions are selectively populated and the thermal regions in which conforma- However, when the samples are incubated at a temperature close tional transformations are observed. The relevant DNA states are shown in to the onset of the exchange exotherm, some residual fraction of the diagram form. Note the unusual exothermic peak Ϸ50 °C, which is reflective original exchange exotherm is detected upon rescan after cooling; of strand exchange between the 2 ⍀-DNAs to form the 2 fully paired duplex evidence for some, but not all, of the ⍀-DNA metastable state states. undergoing conversion
Details
-
File Typepdf
-
Upload Time-
-
Content LanguagesEnglish
-
Upload UserAnonymous/Not logged-in
-
File Pages5 Page
-
File Size-