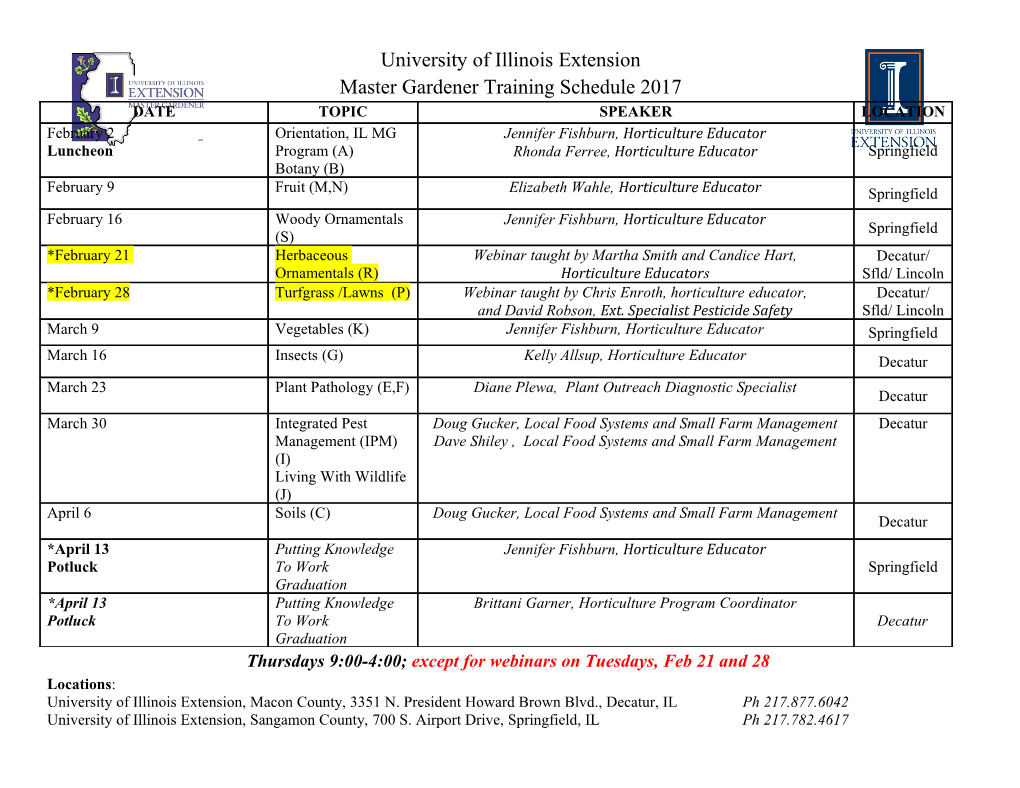
American Mineralogist, Volume 96, pages 682–689, 2011 The fractional latent heat of crystallizing magmas S.A. MOR S E * Department of Geosciences, University of Massachusetts, 611 North Pleasant Street, Amherst, Massachusetts 01003-9297, U.S.A. AB S TR A CT The fractional latent heat of a crystallizing system is simply the latent heat of fusion divided by the total enthalpy. When plotted against temperature, this function displays robust pulses with the successive saturation of each incoming crystal phase. Each pulse endures for tens of degrees, cor- responding to tens to hundreds of thousands of years in large magma bodies. From an original 1963 development by P.J. Wyllie using a synthetic system, I show that the liquidus slopes (degrees per gram of solid produced) decrease discontinuously at the arrival of a new phase, and their inverse, the crystal productivity, undergoes sharp upward pulses at the same time. The overall liquidus slope increases continuously, interrupted by small downward jumps, with the evolution of the multicomponent melt. The crystal productivity pulses feed the fractional latent heat pulses, which dominate the crystalliza- tion history of a melt. These elementary relationships govern the near-solidus growth of dihedral angles in cumulates as they relate to the liquidus events. The latent heat ordinarily approximates to about 80% of the total enthalpy budget, but jumps to 100% (by definition) when solidification oc- curs by isothermal adcumulus growth and approaches 100% when mafic phases such as augite and Fe-Ti oxides are over-produced (relative to their equilibrium saturation) in layered intrusions. The feedback of latent heat to a self-regulating cooling history of large magma bodies is deduced here in principle. The overall results help to clarify the crystallization history of mafic magmas. In particular, they support the solidification of floor cumulates by interchange with parent magma and without the help of compaction. Keywords: Latent heat, enthalpy, solidification, mafic magma, layered intrusions, crystal produc- tivity, Skaergaard intrusion INTRODUCT I ON occurs or negative when crystallization occurs. In common prac- This paper is a study of the heat effects encountered during tice we may use the term latent heat of fusion without regard to the crystallization of a mafic magma. It begins with a simple sign and understand the process from the context. The fractional synthetic ternary system, diopside-anorthite-albite, (Bowen latent heat is the enthalpy of fusion evolved during crystallization 1915) within which the crystallizing melt at first runs away divided by the total enthalpy of the system (which is the sum of from a single-crystal phase, here diopside. From the path thus the sensible heat, i.e., the heat capacity multiplied by the change followed, the crystal productivity is calculated as the mass of in temperature, and the latent heat). Over the history of crystal- diopside per degree of temperature. Then the liquid is followed lization, within a system of constant bulk composition cooling along the cotectic with plagioclase, and the crystal productivity at steady state, the fractional latent heat is released in durable calculated again. It turns out that the crystal productivity jumps pulses that coincide with the arrival of each new phase on the discontinuously to a much higher value when all three phases are liquidus. In large magma bodies, these pulses may endure for present. Wyllie (1963) discussed this sequence in terms of the thousands of years and they have, therefore, the ability to prolong changes in liquidus slope from steep to shallower as the number the textural maturation of the cumulate near the solidus. It is this of solid phases increases. He also emphasized two important periodic effect of the durable release of latent heat that exactly features of such multiphase equilibria. First, although the slope correlates with the jumps in dihedral angle with each incoming of the liquidus at first flattens onto what he called shelves, within liquidus phase in the Skaergaard intrusion (Holness et al. 2007) the shelf history the liquidus steepens again. This tendency is and therefore defines a cause-and-effect of textural maturity. repeated in successive shelves. Second, he emphasized that: “the This interpretation of the Skaergaard dihedral angles has been latent heat of fusion is an important item in the thermal budget” hotly contested (McBirney et al. 2009) and as strongly defended (italics in the original). That emphasis is the goal of this paper, (Holness et al. 2009, including the present author), and one pur- in which the path from the crystal productivity to the fractional pose of this contribution is to clarify the origin of the pulses of latent heat is derived. latent heat that may have been obscure in Holness et al. (2007, The heat absorbed in a chemical reaction is taken as positive 2009). However, the results of this exercise also ramify to issues and hence the enthalpy of fusion is taken as positive when fusion of isothermal solidification by chemical exchange (adcumulus growth) and of compaction in igneous cumulates. The magma types considered here are primarily those that * E-mail: [email protected] crystallize the sequence olivine-plagioclase-augite-(ilmen- 0003-004X/11/0004–682$05.00/DOI: 10.2138/am.2011.3613 682 MORSE: FRACTIONAL LATENT HEAT 683 1 ite)-magnetite-apatite (and possibly ternary feldspar) such as the liquid evolves. At the end of stage 2, all of the remaining those at Skaergaard and the Kiglapait intrusion (Morse 1969) liquid from stage 1 has produced crystals of plagioclase and and many other layered intrusions (Cawthorn 1996), but also, diopside, again with a drop of 35 °C, but with much greater and interestingly, some varieties of primitive MORB as in the crystal productivity (2.37 g/°C). The first slope (35/17.2 = 2.0 Ghiorso (1997) MELTS exercise from which this treatment of °C/g) has now given way to the shallower mean slope (35/82.8 the fractional latent heat is taken. = 0.42 °C/g), permitting a much greater yield of crystals per degree, and hence, a much greater ratio of latent heat to sensible THE WYLL I E S EQUENCE IN DI-AN-AB heat released. Equilibrium crystallization Fractional crystallization The quasi-ternary system Di-An-Ab (Fig. 1) furnishes a In the more interesting case of fractional crystallization, the straightforward opportunity to grasp the basic principles of liquid will continue to move along the cotectic to the Di-Ab Wyllie’s (1963) discussion of a sequence of slopes and shelves. sideline, upon which it leaves the ternary system into quater- The figure shows a simple equilibrium crystallization path from nary space. One may ignore its further history and leave the the diopside field to the plagioclase cotectic and then along the plagioclase composition stalled at An9. Accordingly, consider cotectic until the total solid composition has reached the bulk only the path of the liquid to the sideline and the crystals to their composition, at which point by definition, the liquid is consumed. limiting composition. The T-X plot (Fig. 2a) illustrates the liquid The two segments of this path are labeled 1 and 2. In segment and crystal paths as well as the total solid composition path.2 1, diopside crystals have formed and the liquid has been driven The calculation gives a residue of 13% liquid at the sideline to the cotectic over a range of temperature from 1270 to 1235 and hence, a total fraction solidified equal to 0.87, or 87%. It is °C. By means of the lever rule, 17.2% of the system has now of critical importance to note the steepening of the liquidus as crystallized as diopside, so 100Fs = 17.2, where Fs is the fraction it approaches the limit. solid. From these relations the mean crystal productivity has been No single value of crystal productivity is of interest with re- 0.49 g of diopside per degrees celsius. gard to this fractional crystallization path but instead, a sampling At this point, the liquid turns sharply away from the Di path of 18 individual stages from the fractionation output is more and runs along the cotectic (stage 2 of the process), crystalliz- informative, as shown in Figure 2b. Productivity is calculated ing large quantities of plagioclase along with diopside. Ideally from the Rayleigh fractionation output as the stepwise difference the plagioclase crystals react perfectly to maintain pervasive in the fraction solidified divided by the stepwise temperature equilibrium with liquid, adjusting their entire composition as difference. In the figure, it is clear that there is a discontinuous jump in productivity when the liquid reaches saturation with Di 1391.5 plagioclase, and that this new maximum is followed by a steep decline over a range of 50 °C to values below the pre-cotectic level. One may presume that this behavior is characteristic of 1350 all such new saturations in crystal species that a fractionating magma encounters. 2 1 100 Fs = 82.8 100 F = 17.2 STUD I E S U SI NG MELTS ON MORB 1300 s T = 35deg T = 35deg xl Prod = 2.37 g/deg xl Prod = 0.49 g/deg Thermal slopes of evolving magmas 1 Wyllie (1963, p. 209) emphasized abundant evidence that 1250 the liquidus slopes within the shelves of evolved magmas tend 2 to become steeper all the way to a limit. Since plagioclase is 1300 1200 1350 a ubiquitous phase in such an evolution of a common mafic 1250 1400 1 1133 1450 This would be Bowen’s (1913) hypothetical “perfect equilib- 1200 1500 1150 rium.” In reality we know that this equilibration is impossible Ab An 1100 Weight percent 1553 in a dry system because it would take all of geologic time to equilibrate a 1 mm grain of plagioclase internally at 1000 °C FI GURE 1.
Details
-
File Typepdf
-
Upload Time-
-
Content LanguagesEnglish
-
Upload UserAnonymous/Not logged-in
-
File Pages8 Page
-
File Size-