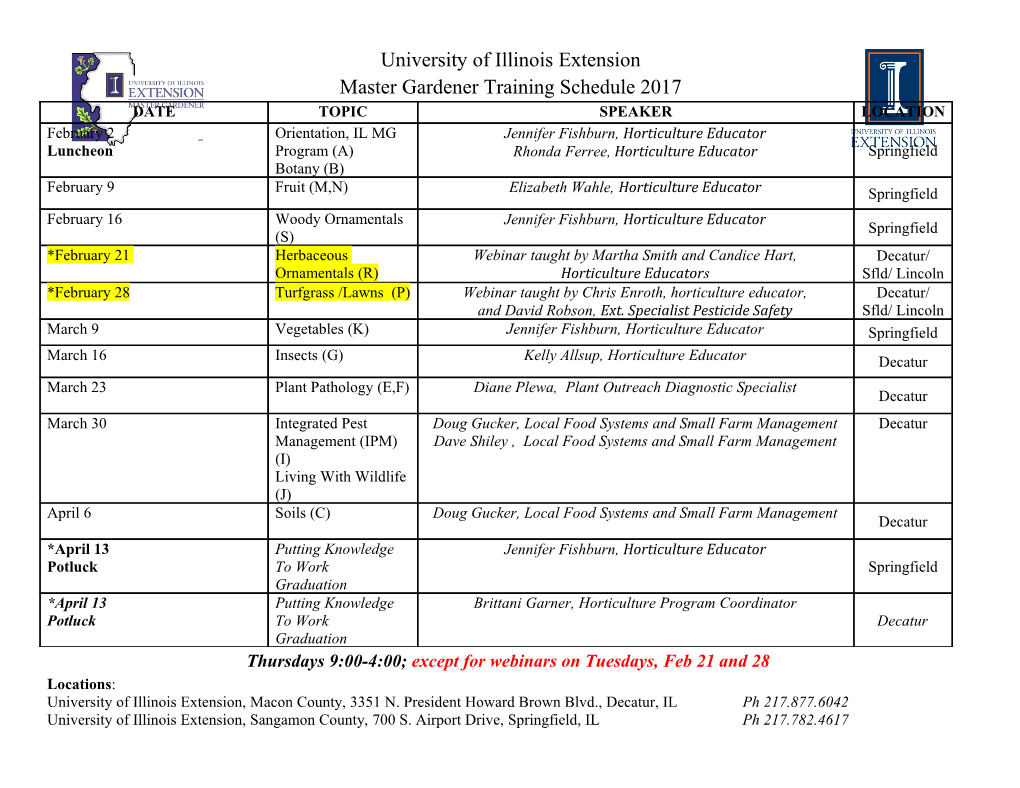
Nitric oxide synthase domain interfaces regulate PNAS PLUS electron transfer and calmodulin activation Brian C. Smitha, Eric S. Underbakkea, Daniel W. Kulpb, William R. Schiefb, and Michael A. Marlettaa,1 Departments of aChemistry and bImmunology and Microbial Science, The Scripps Research Institute, La Jolla, CA 92037 Edited by Robert T. Sauer, Massachusetts Institute of Technology, Cambridge, MA, and approved August 7, 2013 (received for review July 14, 2013) Nitric oxide (NO) produced by NO synthase (NOS) participates in subdomain then donates this electron to the heme domain. How- diverse physiological processes such as vasodilation, neurotrans- ever, in the input state, FMN is buried within the rest of the mission, and the innate immune response. Mammalian NOS reductase domain and inaccessible to the heme domain (2, 4). isoforms are homodimers composed of two domains connected Therefore, for electron transfer from FMN to heme the FMN by an intervening calmodulin-binding region. The N-terminal subdomain must undergo a largely uncharacterized conforma- oxidase domain binds heme and tetrahydrobiopterin and the tional change (∼70 Å) in which the FMN subdomain interacts with arginine substrate. The C-terminal reductase domain binds FAD the heme domain (3, 5). This FMN subdomain conformation and FMN and the cosubstrate NADPH. Although several high- is known as the output state (Fig. 1B). Understanding the mech- resolution structures of individual NOS domains have been anism of electron transfer from FMN to heme is particularly reported, a structure of a NOS holoenzyme has remained elusive. important as this electron transfer step is rate limiting in NOS Determination of the higher-order domain architecture of NOS is catalysis (3). essential to elucidate the molecular underpinnings of NO forma- Calmodulin is required for NOS catalyzed NO formation. tion. In particular, the pathway of electron transfer from FMN to Calmodulin binding to the helix connecting the heme and re- heme, and the mechanism through which calmodulin activates this ductase domains releases the FMN subdomain from the input – electron transfer, are largely unknown. In this report, hydrogen state (3). However, the extent of calmodulin interaction with the deuterium exchange mass spectrometry was used to map critical heme domain remains unclear. Establishing whether or not cal- NOS interaction surfaces. Direct interactions between the heme modulin forms a direct interface with the heme domain is nec- BIOCHEMISTRY domain, the FMN subdomain, and calmodulin were observed. essary to determine the mechanism through which calmodulin fi These interaction surfaces were con rmed by kinetic studies of activates the rate-limiting electron transfer to the heme. Cur- fi – site-speci c interface mutants. Integration of the hydrogen deu- rently, NOS structural information is limited to truncations terium exchange mass spectrometry results with computational encompassing the iNOS heme domain (6, 7), the nNOS re- docking resulted in models of the NOS heme and FMN subdomain ductase domain (NAPDH, FAD, and FMN subdomains) (4, 8), bound to calmodulin. These models suggest a pathway for elec- or the iNOS FMN subdomain bound to calmodulin (9). The tron transfer from FMN to heme and a mechanism for calmodulin protein interfaces constituting the output state of the FMN activation of this critical step. subdomain remain unknown (Fig. 1B), and the precise role of calmodulin in NOS catalysis is poorly understood. iNOS | NO signaling | flavin | hemoprotein Previous studies have attempted to define interactions be- tween the heme and reductase domains using computational itric oxide (NO) has several essential functions in mamma- docking (9–11). However, selecting the catalytically relevant Nlian physiology. NO produced by the neuronal and endothe- structure from many alternative models is difficult without direct lial nitric oxide synthase isoforms (nNOS and eNOS, respectively) experimental evidence delineating the protein–protein interfaces initiates diverse signaling processes including vasodilation, myo- between the heme domain, FMN subdomain, and calmodulin. cardial function, and neurotransmission (1). The eNOS and nNOS isoforms are constitutively expressed and their activity Significance responds to intracellular calcium concentrations. The inducible NOS isoform (iNOS) is transcriptionally controlled and pro- duces NO as a cytotoxin at sites of inflammation or infection. The biological role of nitric oxide (NO) in mammalian physiol- Aberrant NO signaling contributes to a variety of diseases in- ogy is now well established as a signaling molecule in the cluding stroke, hypertension, and neurodegeneration (2). cardiovascular and nervous systems and as a chemical compo- Mammalian NOS isoforms are homodimeric and composed of nent in the host response to infection. NO is synthesized by the two principal domains: the N-terminal oxidase domain and enzyme NO synthase (NOS). The structure of the entire NOS C-terminal reductase domain, which are connected by an inter- enzyme has not been solved, but the structure of isolated domains has been reported. In this study, we use a mass vening calmodulin (CaM) binding region (Fig. 1A). The N-terminal spectrometry approach (hydrogen–deuterium exchange) to oxidase domain contains the heme and tetrahydrobiopterin cofac- find interaction surfaces of the native protein. These results tors and the binding site for the substrate arginine. The reductase were then used to generate NOS models, which revealed in- domain is further divided into the FMN-binding subdomain and teraction surfaces that mediate NOS activity. These interacting the FAD/NADPH-binding subdomains. This array of cofactors surfaces provide insight into the conformational changes and works in concert to catalyze the conversion of arginine to the residues necessary for regulating NOS activity. intermediate N-hydroxyarginine and, ultimately, citrulline and NO. NADPH and oxygen are consumed in the process. During Author contributions: B.C.S., E.S.U., D.W.K., W.R.S., and M.A.M. designed research; B.C.S., catalysis, electrons are shuttled from the reductase domain of E.S.U., and D.W.K. performed research; B.C.S., E.S.U., D.W.K., W.R.S., and M.A.M. analyzed one monomer to the heme domain of the opposite monomer in the data; and B.C.S., E.S.U., D.W.K., W.R.S., and M.A.M. wrote the paper. homodimer (Fig. 1B) (1, 3). Electron transfer is initiated by two- The authors declare no conflict of interest. electron reduction of FAD by NADPH. FMN then accepts an This article is a PNAS Direct Submission. electron from FAD to FMN through the interaction of the FMN 1To whom correspondence should be addressed. E-mail: [email protected]. subdomain with the FAD subdomain. This FMN subdomain in- This article contains supporting information online at www.pnas.org/lookup/suppl/doi:10. teraction state is known as the input state (Fig. 1B). The FMN 1073/pnas.1313331110/-/DCSupplemental. www.pnas.org/cgi/doi/10.1073/pnas.1313331110 PNAS Early Edition | 1of10 Downloaded by guest on September 30, 2021 heme-FMN:CaM) have been used to study electron transfer A Heme domain Reductase domain between FMN and heme and therefore are excellent models of CaM the FMN output state (14, 15). N Fe(III) FMN FAD NADPH C Mapping Interactions of the iNOS Heme Domain. HDX-MS was used 66 498 709 724 1144 to compare the solvent accessibility of iNOS heme and iNOS - C B Input state e heme-FMN:CaM (Fig. 1 ). Slower exchange rates in iNOS heme-FMN:CaM were expected in regions of the heme domain NFHPDA FAD FMN Fe(III) Fe(III) MNPDANDAF H that interact with the FMN subdomain or calmodulin. Full time courses for each unique peptide are shown in Fig. S1. Differ- - - Output state e e ences in percent exchange rate between iNOS heme and iNOS C CaM heme-FMN:CaM were mapped to the primary iNOS heme do- main sequence (Fig. 2A). Several regions of the iNOS heme Fe(III) FMN iNOS Heme-FMN:CaM domain exhibited significant slowing of deuterium exchange when attached to the FMN:CaM module. No regions exhibited Fe(III) iNOS Heme faster exchange in iNOS heme-FMN:CaM. Whereas decreases in dynamics may also contribute to the observed slower exchange rates, the HDX-MS data are consistent with the observed effects CaM in the heme domain being primarily due to burial of protein– FMN iNOS FMN:CaM protein interaction interfaces. Mapping the regions displaying exchange rate perturbations to a crystal structure of the murine iNOS heme domain (6) revealed a clear interaction surface that Fig. 1. Mammalian nitric oxidase synthases. (A) Mammalian nitric oxide spanned the iNOS heme domain dimer interface (Fig. 2B). synthase domain organization. (B) Nitric oxide synthase electron transfer Importantly, this interaction surface is of sufficient size to ac- pathway. In the input state, the FMN subdomain interacts with the FAD subdomain allowing electron transfer to occur between the flavin cofactors. commodate interactions with both the iNOS FMN subdomain In the output state, the FMN subdomain interacts with the heme domain and calmodulin. allowing electron transfer between the FMN and heme. (C) iNOS constructs used in this study. iNOS heme-FMN:CaM consists of residues 66–709 bound to Mapping Interactions of the iNOS FMN Subdomain with the Heme calmodulin. The hyphen in heme-FMN:CaM indicates the native covalent Domain. To elucidate the interaction surfaces between the FMN linkage of the heme domain and FMN subdomain and the colon indicates the subdomain and the heme domain, HDX rates of iNOS FMN:CaM noncovalent interaction of calmodulin with iNOS heme-FMN. iNOS heme were compared with iNOS heme-FMN:CaM (Fig. 1C). Mapping consists of residues 66–498. iNOS FMN:CaM consists of residues 499–709 the differences in exchange rate to the iNOS FMN subdomain bound to calmodulin. All residue numbering refers to residues in murine iNOS. primary sequence showed two regions with slowed exchange in iNOS heme-FMN:CaM (Fig.
Details
-
File Typepdf
-
Upload Time-
-
Content LanguagesEnglish
-
Upload UserAnonymous/Not logged-in
-
File Pages10 Page
-
File Size-