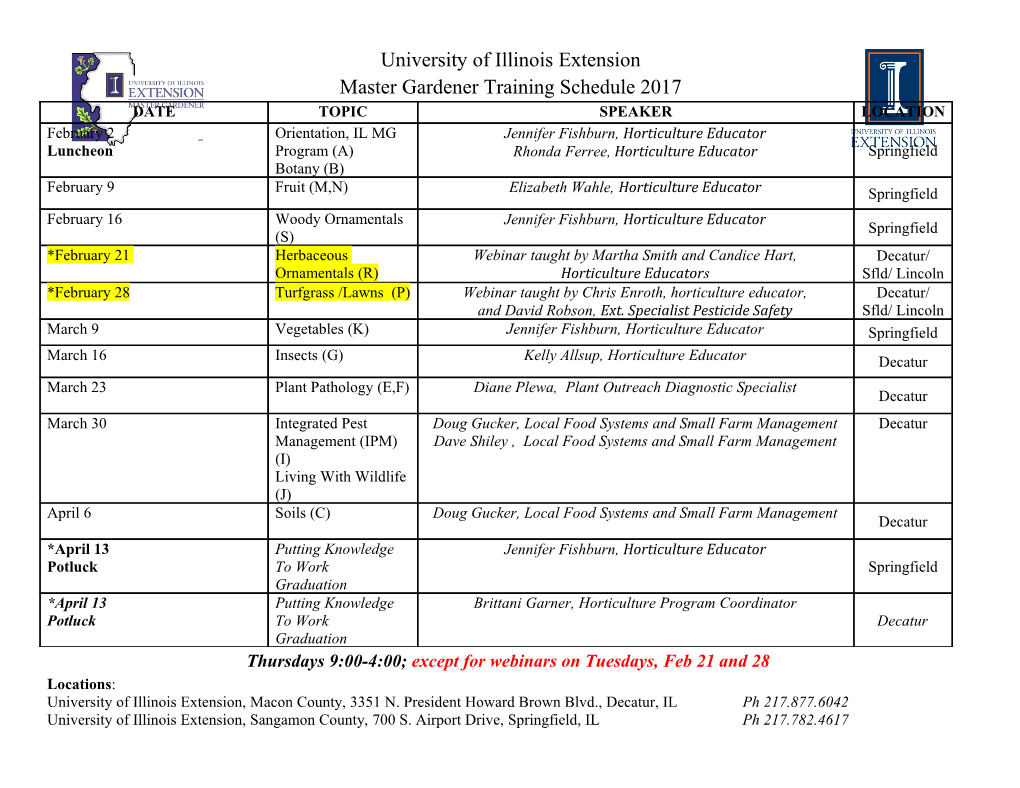
Genetica 115: 49–63, 2002. 49 © 2002 Kluwer Academic Publishers. Printed in the Netherlands. Transposable elements and the evolution of genome size in eukaryotes Margaret G. Kidwell Department of Ecology and Evolutionary Biology, The University of Arizona, Tucson, AZ 85721, USA (Phone: (520) 621-1784; Fax: (520) 621-9190; E-mail: [email protected]) Key words: genome size, molecular evolution, transposable element Abstract It is generally accepted that the wide variation in genome size observed among eukaryotic species is more closely correlated with the amount of repetitive DNA than with the number of coding genes. Major types of repetitive DNA include transposable elements, satellite DNAs, simple sequences and tandem repeats, but reliable estimates of the relative contributions of these various types to total genome size have been hard to obtain. With the advent of genome sequencing, such information is starting to become available, but no firm conclusions can yet be made from the limited data currently available. Here, the ways in which transposable elements contribute both directly and indirectly to genome size variation are explored. Limited evidence is provided to support the existence of an approximately linear relationship between total transposable element DNA and genome size. Copy numbers per family are low and globally constrained in small genomes, but vary widely in large genomes. Thus, the partial release of transposable element copy number constraints appears to be a major characteristic of large genomes. Introduction Bearing in mind that genome sequence data are mostly incomplete and limited to a few species, the In contrast to the relatively narrow range of ge- purpose of this paper is to initiate a broad scale survey, nome sizes in prokaryotes, eukaryotic species vary involving both animals and plants, into the extent to more than 200,000-fold in the size of their genomes which transposable elements determine genome size. (Gregory, 2001). No single factor, such as number A major aim will be to inquire whether or not there is of coding genes or degree of organismal complexity evidence for any broad generalizations concerning the is closely correlated with genome size, giving rise to types and copy numbers of transposable elements that the so-called ‘C-value paradox’. Today, it is gener- distinguish small from larger genomes. ally accepted that differential amounts of non-coding, repetitive, DNA account for a major fraction of euka- ryotic genome size variation, thus providing a partial Transposable elements and genome evolution explanation of the paradox (Gregory & Hebert, 1999; Petrov, 2001). However, a whole set of questions re- Transposable elements are potent mutagenic agents main unanswered, including the relative contributions with the potential to produce a wide array of changes of various types of repetitive DNA, such as transpos- in the genomes of their hosts (Kidwell & Lisch, 2000; able elements, satellite DNAs and simple sequence 2001). The range of ‘mutations’ induced by trans- repeats to the variation in genome size. It is also posable element activity extends from modifications unclear how genome sizes are contained or reduced. in the size and arrangement of whole genomes to These questions are highly complex with no simple substitutions, deletions, and insertions of a single nuc- answers likely to be found because the evolution- leotide. In addition to their ability to increase genome ary forces shaping genome size vary widely among size following transposition, transposable elements in- different organisms (Petrov, 2001). duce chromosomal rearrangements such as deletions, 50 duplications, inversions, and reciprocal translocations. be interesting to determine to what extent transposi- This activity provides the potential for small- and tion is associated with one or both of these patterns of large-scale genome reorganization, amplification, and genome size increase. reduction. Transposable elements have been found at the Transposable element classification origin of numerous types of chromosomal rearrange- ments in many species (e.g., Lim & Simmons, 1994; Most transposable elements can be assigned to two Caceres et al., 1999) Increases in transposable ele- main classes (see Table 1), according to their mech- ment copy number following transposition have also anism of transposition (Finnegan, 1989; Capy et al., been directly implicated in significant genome size ex- 1997). Both autonomous and nonautonomous mem- pansions over a relatively short period of evolutionary bers are found in many element families of both time (e.g., a doubling of the maize genome during the classes. Autonomous elements are able to catalyze last few million years (SanMiguel et al., 1998)). Trans- their own transposition, while nonautonomous, ele- posable elements are undoubtedly responsible for a ments depend on autonomous elements from the same significant proportion of the observed karyotypic vari- family for transposition. Class I elements are mem- ation among many groups. Reliable estimates of the bers of the larger group of Retroid agents that also fraction of all rearrangements accounted for by trans- includes retroviruses (McClure, 1999). They use an posable elements are currently not available in any RNA-mediated mode of transposition and encode a re- species, but are urgently needed. These elements may verse transcriptase (RT). These elements are amplified also produce mutations when they excise imprecisely, by the transposition process and have a high potential leaving either no identifying sequence, or only small for increase in copy number. They are divided into two ‘footprints’ of their previous presence. A major prob- subclasses, the retrotransposons that are characterized lem of identification arises because of rapid divergence by direct, long terminal repeats (LTRs), and the retro- of nonautonomous elements and the origin of many posons that lack terminal repeats. Here these elements ancient sequences may be difficult, if not impossible, will be referred to as LTR retroelements (or LTR retro- to identify. transposons) and non-LTR retroelements, respectively. The LTR retroelements include four distinct groups (Malik et al., 2000), Ty1-copia, BEL, DIRS,andTy-3 Gradual changes versus quantum jumps gypsy, that are broadly distributed in many animals and plants. The non-LTR retroelements include the long A useful distinction has been made between global interspersed nuclear elements (LINEs) and the short and local forces that act to modulate genome size interspersed nuclear elements (SINEs). (Petrov, 2001). It is expected that relatively fast Many Class II elements, the transposons (sensu genome expansion will be modulated by the ac- strictu), use a DNA-mediated mode of ‘cut and paste’ tion of global forces. Both global and local forces transposition. However, it was recently shown that a would be expected to contribute to more gradual number of eukaryotic Class II elements, called He- changes. Transposable elements, along with poly- litrons, have probably propagated by a mechanism ploidization, are good examples of global forces for similar to rolling-circle (RC) transposition in proka- expansion, whereas expansion of microsatellites, het- ryotes (Kapitonov & Jurka, 2001). Helitrons tend to erochromatin, or various types of tandemly repeated be large (Table 1) and, surprisingly, constitute as much sequences, represent local forces. as 2% of the genome of Arabidopsis thaliana and Several interesting patterns of genome size variab- Caenorhabditis elegans (Kapitonov & Jurka, 2001). ility have been observed. For example, among birds, The ‘cut and paste’ Class II elements include the hAT mammals and teleost fish, genome size variation is (hobo, Activator, Tam-3) superfamily of elements, the relatively small, suggesting that changes occur by mariner-like superfamily of elements, the P elements, gradual accretion of small blocks of DNA (Gregory & and the MuDR elements. Although the miniature in- Hebert, 1999). However, the large variation in genome verted repeat transposable elements (MITEs) and the size observed among invertebrates and plants suggest foldback (FB) elements were earlier assigned to a third that quantum jumps make an important contribution class (Capy et al., 1997), subsequent evidence strongly and this has been well documented in some plants suggests that MITEs are members of the Class II ele- (e.g., maize SanMiguel et al., 1996, 1998). It will ments (Le et al., 2000; Turcotte et al., 2001). In 51 Table 1. Characteristics of some widely distributed types of transposable elements Class Subclass Superfamily Family examples Approximate size range (bp) I. Retroelements LTR retro- Ty1-copia Opie-1 in maize 3000–12,000 RNA-mediated transposons BEL Cer7 in 3000–20,000 elements C. elegans DIRS-1 DIRS-1 in ∼5000 Dictyostelium Ty3-gypsy Gypsy in 5000–14,000 Drosophila Non-LTR LINEs LINE-1 in 1000–7000 retroposons humans; I element in Drosophila SINEs Alu in humans 100–500 II. Transposons Cut and paste mariner-Tc1 Tc1 in 1000–2000 DNA-mediated transposition C. elegans; elements DDE signature mariner in present Drosophila Mu Mu in maize; 400–20,000 MULEsin Arabidopsis MITEs Tourist in 100–500 maize Cut and paste hAT hobo in 500–4600 transposition Drosophila; DDE signature Ac in maize; absent Tam-3 in Anthirinhum PPin Drosophila Rolling circle Helitrons Helitrons in 5500–17,500 (RC) transposition A. thaliana, O. sativa,and C. elegans Unclassified Foldback Galileo in Large, but D. buzzatii highly variable Mini-me
Details
-
File Typepdf
-
Upload Time-
-
Content LanguagesEnglish
-
Upload UserAnonymous/Not logged-in
-
File Pages15 Page
-
File Size-