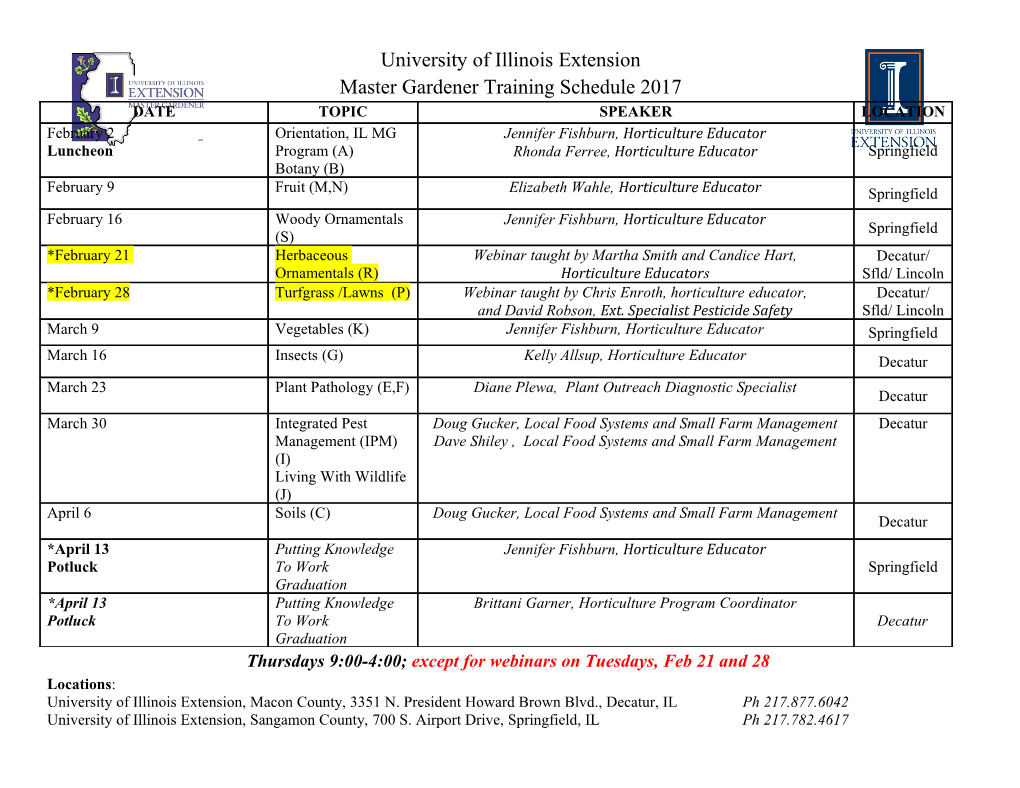
IAWA Journal, Vol. 28 (4), 2007: 445-455 STRUCTURAL CHANGES IN PRIMARY LENTICELS OF OLEA EUROPAEA AND CERCIS SILIQUASTRUM DURING THE YEAR D. Kalachanis and G.K. Psaras Section ofPlant Biology, Department ofBiology, University ofPatras, Patras 265 00, Greece [E-mail: [email protected]] SUMMARY The structure of primary lenticels of the Mediterranean evergreen Olea europaea and the winter deciduous species Cercis siliquastrum was in­ vestigated during the year using scanning electron, conventional bright­ field and epi-fluorescence microscopy. It was revealed that lenticels of O. europaea do not undergo significant structural changes over this time period. The filling tissue of O. europaea lenticels consists of fully-suber­ ized cells that form small intercellular spaces. The air-exposed filling cells are replaced during spring and early summer by new early-suber­ ized cells. Further notable structural modifications during the year were not observed. By contrast, lentice1sof C. siliquastrum possess a closing layer of suberized cells delimiting an underlying mass of non-suberized filling cells. During the period of high metabolie activity of the plant, i.e. during spring and early summer, the suberized closing layer is rup­ tured from the pressure exerted by the newly formed underlying cells. During late summer a new closing layer is formed, delimiting again the non-suberized underlying filling cells during winter. The possible role of lenticels in the gas exchange process is discussed. In both species the shade-adapted parenchyma cells of the cortex beneath lenticels shows bright red auto-fluorescence of chlorophyll, a phenomenon that is not yet fully understood. Key words: Functional anatomy, lenticel, seasonal change, Cercis sili­ quastrum, Olea europaea. INTRODUCTION The periderm is a system of protective tissues that replaces the epidermis when the plant axis increases in thickness by secondary growth. The formation of a periderm is a common phenomenon in sterns and roots of gymnosperms and dicotyledons. Periderm consists of three tissues: the phellogen, which is a secondary meristem, the phellern, which is produced by the phellogen outwards, and the phelloderm, a parenchyma-like tissue that develops inwards. Lenticels are structures of the periderm characterized by loosely arranged cells termed filling cells. The lenticel phellogen is more active than the rest of the phellogen and produces phelloderm cells inwards and filling tissue Associate Editor: Imogen Poole Downloaded from Brill.com10/06/2021 10:48:16AM via free access 446 IAWA Journal, Val. 28 (4), 2007 outwards. The epidermis is ruptured by the lenticel filling tissue, which then protrudes above the epidermis surface. The exposed filling cells usually die and are replaced by new ones produced by the lenticel phellogen. In some species layers of loose filling tissue alternate with layers of compact tissue called closing layers. Whether the cells of the filling tissue are suberized or non-suberized combined with the presence or absence of closing layers has led to the classification of the lenticels. In gymnosperms, the filling cells are suberized and similar to those of the rest of the phellern except for the presence of intercellular spaces among them. In dicotyledons three types oflenticels can be distinguished (Esau 1965). In the first type (Liriodendron­ Magnolia-Populus-Pyrus) the filling cells, although having intercellular spaces, are suberized and the tissue is rather compact. A thin-walled tissue may be succeeded by a thick-walled tissue annually. In the second type (Quercus-Fraxinus-Sambucus-Tilia) more or less loose and non-suberized filling tissue alternates with a more compact layer ofsuberized cells. In the third type (Betula-Fagus-Prunus-Robinia), compact and suberized closing layers alternate with loose, non-suberized filling tissue. The closing layers are successively ruptured by new growth. New closing layers are produced each growing season. Because of the presence of intercellular spaces in the tissue of lenticels it has long been presumed that lenticels of woody sterns may functionally substitute for stomata, permitting the passage of air through the periderm. Recent micro-autoradiography experiments indicate that woody stern chlorenchyma ofFraxinus excelsior assimilates extern al 14C02. The areas bordering the lenticels showed the highest assimilation, indicating that 14C02 enters the stern through the intercellular spaces of the lenticels (Langenfeld-Heyser et al. 1996). The permeability of single lenticels of Betula potaninii and Sambucus nigra sterns to water and oxygen has been found to be significantly higher than that ofthe phellern areas lacking lenticels (Groh et al. 2002; Lendzian 2006). It is important to note that Mancuso and Marras (2003) have shown that the main pathway of the oxygen supply to parenchymatous cells in the sapwood of Olea europaea trees is not by radial diffusion via lenticels but through the transpiration stream. Pfanz et al. (2002) proposed an additional role for the lenticels. They suggested that lenticels facilitate sunlight permeance, resulting in effective corticular photosynthesis. In a sub­ sequent study, however, Manetas and Pfanz (2005) found light transmittance of lenticel regions to be considerably lower than that of non-lenticular regions. In the past lenticels were considered to be static structures. Recently, however, this idea has been revised. Wutz (1955), and more recently Rosner and Kartusch (2003), have reported that lenticels undergo structural modifications during the year. According to Lendzian (2006), gas exchange through phellern is regulated by long-term structural modifications. Thus, structural changes of the lenticels may reflect long-term regulation mechanisms whose function is yet to be clarified. The aim of this work was to investigate the structural modifications of the primary lenticels of the Mediterranean evergreen Olea europaea and the winter deciduous Cercis siliquastrum sterns during a year. These data contribute to a better understanding ofthe role that lenticels play in the regulation mechanisms of stern metabolism. Downloaded from Brill.com10/06/2021 10:48:16AM via free access Kalachanis & Psaras - Structural changes in lenticels 447 MATERIALS AND METHODS Plant material Olea europaea L., an evergreen plant typical of the Mediterranean ecosystems and Cercis siliquastrum L., a deciduous tree, were the focus of this study. Sterns were collected from plants growing wild on the Patras University campus (38° 14' N, 21° 44' E, alt. 125 m), in north-west Peloponnisos, Greece. Sterns at three developmen­ tal stages, i.e. one-, two- and five-to-six-year old were sampled from three individuals of both species. Sampling was undertaken monthly from November 2004 to June 2006. Fresh plant material and epi-fiuoresence microscopy Fresh plant material was studied under a stereoscope (Stemi 2000-c, Zeiss, Ober­ kochen, Germany). Toestimate lenticel surface area, micrographs were digitally record­ ed using a colour video camera (Sony, SSC-DC58AP, Japan). Cross sections, 40 um thick, of all developmental stages were made using a Leica sliding microtome (Leica SM 2000 R, Nussloch, Germany). Sections were stained and examined with an epi-fluorescence microscope. Suberin was detected using Sudan III, Toluidine blue 0 (Ma & Peterson 2001) or Fluorol yellow 088. The latter sections were examined under epi-fluorescence optics. Fluorol yellow 088 is a fluorochrome that efficiently stains lipids in sections of fresh plant material (Brundrett et al. 1991). After comparing the results of these three staining methods it was decided that the latter gave the most useful results. Thus, micrographs presented here are taken from sections stained with Fluorol yellow 088, using the appropriate filter set (02, Zeiss: exciter filter G365, chromatic beam splitter FT 395 and barrier filter LP420). Chlorophyll auto­ fluorescence was detected using a blue excitation filter set (09, Zeiss: exciter filter 450-490, chromatic beam splitter 510 and barrier filter LP520). The sections were examined with a Zeiss Axioplan microscope (Zeiss, Oberkochen, Germany) equipped with epi-fluorescence optics (RBO 50 W mercury lamp) and recorded using a digital camera (AxioCam MRc 5, Zeiss). Tissue fixation for light and scanning electron microscopy Stern samples from all three developmental stages were carefully cut and fixed in 5 % glutaraldehyde in phosphate buffer at pR 7 at room temperature for 2 h. Tissue was then post-fixed in 1% Os04 at 4 °C and dehydrated in a graded acetone series. For light microscopy, tissue samples were embedded in Durcupan ACM (Fluka, Buchs, Switzerland). Semi-thin sections (1-2 um thick) of plastic embedded tissue made on a Reichert Om-U2 (Wien, Austria) microtome using glass knives were stained with Toluidine Blue O. The sections were examined and digitally recorded as described above. For scanning electron microscopy dehydrated tissue samples were critical point dried, mounted with a double adhesive tape on stubs, sputter coated with gold, and observed with a JEOL 6300 (Japan) scanning electron microscope (SEM). Downloaded from Brill.com10/06/2021 10:48:16AM via free access 448 IAWA Journal, Val. 28 (4), 2007 Downloaded from Brill.com10/06/2021 10:48:16AM via free access Kalachanis & Psaras - Structura l cha nges in lenticels 449 RESULTS Olea europaea Young sterns ofO. europaea are densely covered by peltate trichome s. Primary lenti­ cels are alread y present at the end of the first year of growth along with the formation of periderm. Individuallenticel surface area depends on the stern age and ranges from 0.03 to 0.60 mm-. Together, lenticels occupy 5.4±0.46% ofthe stern surface area. Primary lenticels of O. europaea are circular in surface view (Fig. IA, B). The filling tissue is more or less compact (Fig. IC, 0 ), although small intercellular spaces may be observed in seetions (Fig. 2A- 0). As revealed by histochemical reactions the walls of filling cells are already fully suberized (Fig. 4A , B). As seen under both the SEM and the bright-field micro scope lenticels do not undergo any structural modification durin g the year (cf Fig. IA & Band Fig. 2E & F; winter and summer respectively).
Details
-
File Typepdf
-
Upload Time-
-
Content LanguagesEnglish
-
Upload UserAnonymous/Not logged-in
-
File Pages12 Page
-
File Size-