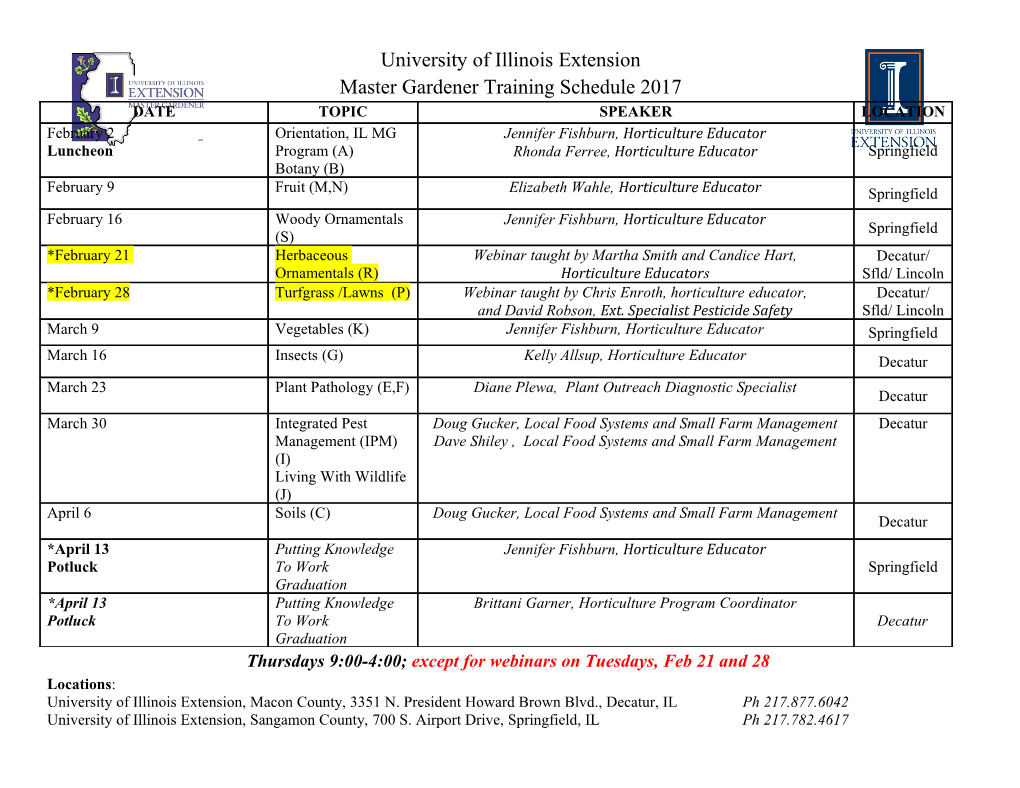
Scott K. Process intensification: An electrochemical perspective. Renewable and Sustainable Energy Reviews (2017) DOI: https://doi.org/10.1016/j.rser.2017.05.189 Copyright: © 2017. This manuscript version is made available under the CC-BY-NC-ND 4.0 license DOI link to article: https://doi.org/10.1016/j.rser.2017.05.189 Date deposited: 13/07/2017 Embargo release date: 10 June 2018 This work is licensed under a Creative Commons Attribution-NonCommercial-NoDerivatives 4.0 International licence Newcastle University ePrints - eprint.ncl.ac.uk Process Intensification: An electrochemical perspective (for Renewable and Sustainable Energy Reviews) Keith Scott, School of Chemical Engineering and Advanced Materials, Newcastle University, NE17RU, UK Abstract This paper provides a review of a range of process intensification methods which have been applied to electrochemical processes and reactions. The electrochemical reactions include those in electrolysers where gas evolution occurs, in metal deposition reactions and reactions occurring in fuel cells and batteries. The process intensification methods include the use of centrifugal force fields, ultrasonics, rotating electrodes and rotating cells which operate with and without the application of magnetic fields. Such devices can be used in principle to carry out electrolysis without the application of an external potential or current. Other methods considered include the use of light. The area of magnetic field induced fluid flow is also discussed which has applications in lab on a chip technologies. The area of process intensification has largely focused a lot on hydrogen generation as a means of providing a clean, sustainable fuel. Keywords: Process intensification, electrochemical cells, electrolysis, magnetic field, rotation, centrifugal field, ultrasound, power generation, hydrogen, fuel cells and batteries 1 1. Introduction Process intensification (PI) may be seen as a generic method or series of tools that seeks to improve process performance in terms of better energy efficiency, lower product or system cost, and better sustainability. As such the application of PI looks to exploit different equipment (reactors, separators, contactors) and apply different methods, such as alternative fluids and phases, extreme operating conditions, multi-functional devices and energy types such as centrifugal fields, ultrasound, solar, light, microwaves, electric fields, plasmas and magnetic fields [1]. Process intensification has been applied to a wide range of reaction systems involving chemical, catalysis and mass transport processes and to a lesser extent electrochemical processes. Electrochemistry is concerned with the transfer of charge, by the movement of ions, in a liquid or solid (or gaseous) phase through which electrochemical transformation of species can be achieved (Fig. 1). Electrochemistry can be used to synthesise materials and chemicals, to generate power and to analyse and detect compounds and components. The applications of electrochemistry are quite diverse and span over a wide range of industries. Improvements in performance of electrochemical technology are targeted at reduced energy consumption, or higher power output (in the case of energy storage devices), increased reaction rates through higher current densities which result in smaller cells and lower cost or the ability to use renewable energy such as wind and solar energy or even biomass or waste in for example bio-electrochemical fuel cells [2]. This article provides a review of strategies that have been applied to improve electrochemical technologies (see Table 1) by applying PI tools, such as centrifugal fields, magnetic fields, light and ultrasound. The review provides examples of where PI has been used advantageously in technologies for synthesis (hydrogen, oxygen, chlorine, organic synthesis, batteries and fuels) and considers the potentials of the approach as well as related limitations. Table 1 2. Electrochemical technologies Electrochemistry is concerned with the transfer of charge, by the movement of ions, in a liquid or solid (or gaseous) phase through which electrochemical transformation of species can be achieved. Electrochemistry can be used to synthesise materials and chemicals, to generate power and to analyse and detect compounds and components. The applications of electrochemistry are quite diverse and span over a wide range of industries, including; energy storage and power generation, synthesis of chemicals and materials, extraction and production of metals, recycling, water purification and effluent treatment, corrosion protection, analysis, sensors and monitors, metal and materials finishing and processing, and semiconductor technology including photo-electrochemistry. Electrochemistry is used in the finishing 2 of many components through the deposition of coatings (metallic, polymer) and through anodising to produce surface oxide films. Electrophoretic painting is used widely in the motor industry for bodywork protection. Other applications are in electropolishing and in electrochemical cleaning, pickling and stripping [3]. An important area for electrochemistry is in sustainable, clean and renewable energy [4]. Renewable energy can be stored in batteries, capacitors or converted into hydrogen for later use. Renewable energy may be used to synthesise chemicals, e.g. from carbon dioxide electrochemical reduction. Solar energy can be used to generate chemicals such as hydrogen and oxygen by photo-electrolysis or in electrosynthesis of chemicals. Energy storage and generation through the application of batteries, capacitors and fuel cells have a common feature; an electrochemical cell featuring two electrodes connected via a conducting electrolyte. The generation of electrical energy within a battery or fuel cell is caused by two “redox” reactions (with a negative free energy) which occur spontaneously or in a capacitor from the liberation of an accumulated charge. Fuel cells are devices for generating electrical power by the continuous supply of a fuel to one electrode and an oxidant to the other electrode. There are a variety of these devices which operate at low or high temperatures. The electrodes in fuel cells are different to those in batteries as they must generally be permeable to gases. In low temperature cells catalytic gas diffusion electrodes are therefore used which are typically a composite structure of electrocatalyst, carbon, hydrophobic binder and a coating. Electrochemical synthesis is used for production of both organic and inorganic chemicals. The two largest industries (in terms of tonnage) are for the combined chlorine and caustic soda (NaOH) production (chlor-alkali) and aluminium electrowinning. These two processes use radically different cell technology, aluminium production being based on molten salt electrolysis at temperatures around 1000 °C, whilst the chlor-alkali industry is based on the electrolysis of aqueous brine solutions at low temperatures around 60 oC. Other inorganic electrochemical processes include the production of hydrogen by water electrolysis, molten salt electrowinning of sodium, lithium and magnesium and electrowinning from aqueous electrolyte of copper, zinc and nickel and a range of compounds such as ozone, hydrogen peroxide, sodium hypochlorite, potassium permanganate, persulphates, etc. [5, 6]. The electrosynthesis of organic chemicals is mainly located in the fine chemical industries where the advantages of this technique have seen somewhere in the region of a hundred or so industrial processes developed. Electrochemical technology is used in relatively few bulk organic chemical syntheses due to the relatively large equipment cost in relation to product value and energy input. In addition the strong presence of heterogeneous catalysis makes competition difficult; especially with a fluctuating 3 electrical energy market. An important exception to this is the production of adiponitrile from acrylonitrile (an intermediate in the production of nylon) [3]. 2.1 Improvement in electrochemical technologies In the electrochemical industries mentioned above a range of strategies have been explored to gain improved performance and better energy efficiencies. These generally introduce methods by which electrocatalysis can be improved by using more active or higher surface area, catalysts and/or by increasing mass transport to electrode surfaces so as to increase reaction rates at lower electrode overpotentials. In the latter case improved mass transport can be achieved by introducing enhanced turbulence through the use for example of turbulence promotors, use of high surface area electrodes or by movement of the electrode structure. Enhanced performance in electrochemical cells can also be realised by modifying conditions of operation, e.g. temperature and pressure, by exploring alternative cell reactions and by using alternative electrolytes and electrodes. 2.1.1. Electrochemical reaction rate, voltage and efficiency The rate at which a species reacts or is produced is defined by Faraday’s Law and can be written as [4]: N I (nF) (mol s-1) (1) Thus for example, in the case of hydrogen formation, Faraday’s Law tells us that 1.0 mole of hydrogen gas (22.4 dm3, at STP) is produced from 2 x 96485 Coulombs (A s) of charge. In electrochemical technology the rate is generally normalised in terms of the cross-sectional area of the electrodes, A, using current density i=I/A. Thus the production capacity, J, is conveniently expressed as [4]: J i CE (nF) mol
Details
-
File Typepdf
-
Upload Time-
-
Content LanguagesEnglish
-
Upload UserAnonymous/Not logged-in
-
File Pages70 Page
-
File Size-