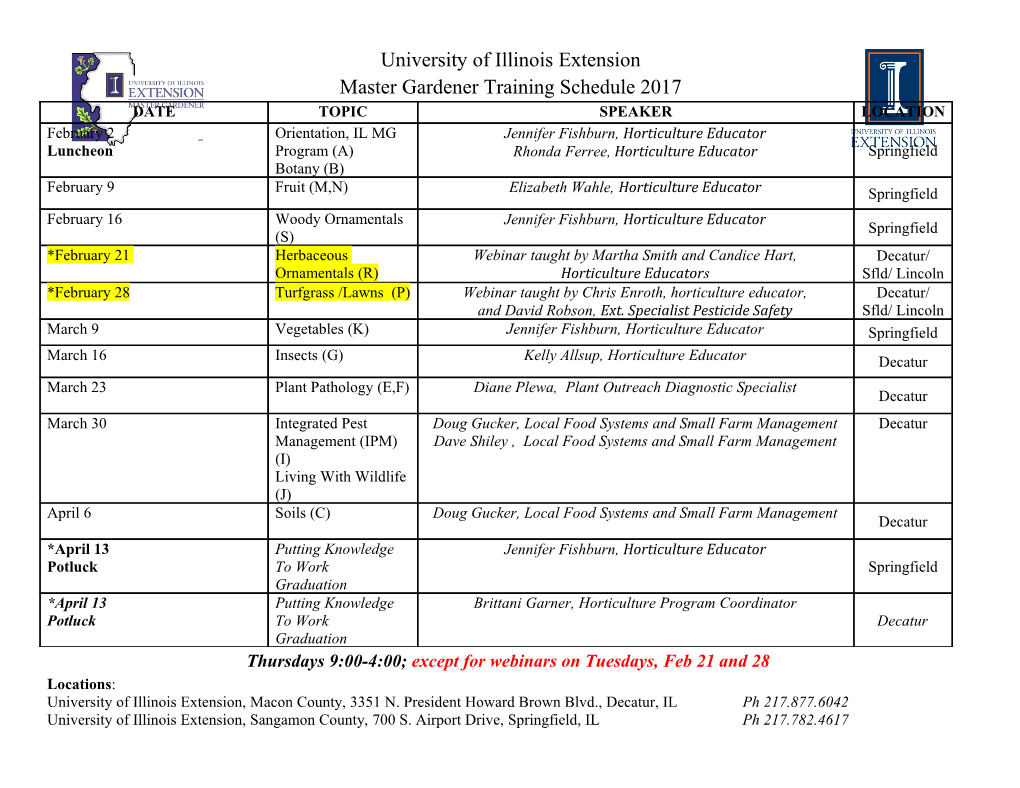
8.08 Anaerobic Metabolism: Linkages to Trace Gases and Aerobic Processes J. P. Megonigal Smithsonian Environmental Research Center, Edgewater, MD, USA M. E. Mines University of Massachusetts Lowell, MA, USA and P. T. Visscher University of Connecticut Avery Point, Groton, CT, USA 8.08.1 OVERVIEW OF LIFE IN THE ABSENCE OF 02 319 8.08.1.1 Introduction 319 8.08.1.2 Overview of Anaerobic Metabolism 319 8.08.1.3 Anaerobic-Aerobic Interface Habitats 321 &08J.4 gy»!az of MekzWism 321 8.08.2 AUTOTROPHIC METABOLISM 322 8.08.2.1 Phototroph (Photolithoautotrophy) Diversity and Metabolism 322 8.08.2.2 Chemotroph (Chemolithoautotrophy) Diversity and Metabolism 324 &0&ZJ Podwayaq/'CC^Fwan'on 325 8.08.3 DECOMPOSITION AND FERMENTATION 325 8.08.3.1 Polymer Degradation 326 8.08.3.1.1 Polysaccharides 327 &08JJ.2 iignin 327 &0&3.2 FenMfnfoA'on 328 &08J.27 AcfiogenaMf 329 8.08.3.2.2 Syntrophy and interspecies hydrogen transfer 329 8.08.4 METHANE 332 8.08.4.1 Methane in the Environment 333 8.08.4.2 Methanogen Diversity and Metabolism 334 8.08.4.3 Regulation of Methanogenesis 335 8.08.4.3.1 O2 and oxidant inhibition 335 &0&4J.2 Mdrwmfa,W;,# 335 8.08.4.3.3 Temperature 336 8.08.4.3.4 Carbon quantity and quality 336 8.08.4.3.5 Plants as carbon sources 337 8.08.4.4 Contributions of Acetotrophy versus Hydrogenotrophy 339 8.08.4.4.1 Organic carbon availability 339 &08.^.^.2 rgmpemfure 341 8.08.4.5 Anaerobic Methane Oxidation 342 8.08.4.6 Aerobic Methane Oxidation 344 317 318 Anaerobic Metabolism: Linkages to Trace Gases and Aerobic Processes 8.08.4.6.1 Methanotroph diversity 344 8.08.4.6.2 Regulation of methanotrophy 346 8.08.4.63 Methane oxidation efficiency 347 8.08.4.7 Wetland Methane Emissions and Global Change 348 8.08.5 NITROGEN 350 8.08.5.1 Nitrogen in the Environment 350 8.08.5.2 Nitrogen Fixation 351 8.08.5.3 Respiratory Denitrification 351 8.08.5.3.1 Denitrifier diversity and metabolism 352 8.08.5.3.2 Regulation of denitrification 353 8.08.5.3.3 Nitrification-denitrification Coupling 355 8.08.5.3.4 Animals and plants 356 &0&5J.5 NzOfWNO/bzej 356 8.08.5.4 Dissimilatory Nitrate Reduction to Ammonium (DNRA) 357 8.08.5.4.1 Physiology and diversity of DNRA bacteria 357 8.08.5.4.2 DNRA versus denitrification 358 8.08.5.5 Alternative Pathways to N2 Production 359 8.08.5.5.1 Anammox 359 8.08.5.5.2 Nitrifier denitrification 361 8.08.5.5.3 Abiotic and autotrophic denitrification 361 8.08.6 IRON AND MANGANESE 362 8.08.6.1 Iron and Manganese in the Environment 362 8.08.6.2 Iron and Manganese Geochemistry 363 8.08.6.3 Microbial Reduction of Iron and Manganese 364 8.08.6.3.1 Metabolic diversity in Fe(III)- and Mn(IV)-reducing organisms 364 8.08.6.3.2 Physical mechanisms for accessing oxides 365 8.08.6.4 Factors that Regulate Fe(III) Reduction 366 8.08.6.4.1 Electron shuttling compounds 366 &0&.6.4.2 Fef/f/JcWafora 367 8.08.6.4.3 Mineral reactivity 367 8.08.6.4.4 Abiotic versus biotic reduction 369 8.08.6.4.5 Separating enzymatic and nonenzymatic Fe(III) reduction 369 8.08.6.4.6 Fe(III) reduction in ecosystems 370 8.08.6.5 Microbial Oxidation of Iron and Manganese 371 8.08.6.5.1 Energetics of Fe(II) and Mn(II) oxidation 371 8.08.6.5.2 Anaerobic Fe(II) oxidation 371 &0&6.5J Aemh'c Fg(7f) ojcidafion 372 &0&&6 /ronCyc/ing 373 8.08.7 SULFUR 374 &0&7.7 W/urGeoc/wmwfry 374 8.08.7.2 Microbial Reduction of Sulfate 375 8.08.7.2.1 Overview of sulfate reduction 375 8.08.7.2.2 Metabolic diversity 376 8.08.7.3 Taxonomic Considerations 377 8.08.7.4 Sulfate-reducing Populations 378 8.08.7.5 Factors Regulating Sulfate Reduction Activity 379 8.08.7.5.1 Sulfate-reducing activity 379 &0& 7.5.2 Tempenzfurg 380 &0&7.5J Carbon 380 8.08.7.5.4 Sulfate and molecular oxygen concentrations 381 8.08.7.6 Microbial Reduction of Sulfur 381 8.08.7.7 Disproportionation 382 &0&7.& W/ur Gases 383 &0&. 7.8.7 gyifwgg»si#(k 383 &0& 7.8.2 MefAyW/Mea 383 8.08.7.8.3 Carbonyl sulfide and carbon disulfide 385 8.08.7.9 Microbial Oxidation of Sulfur 386 8.08.7.9.1 Colorless sulfur bacteria 386 8.08.7.9.2 Single-cell colorless sulfur bacteria 387 8.08.7.9.3 Filamentous colorless sulfur bacteria 388 8.08.7.9.4 Anoxyphototrophic bacteria 388 8.08.7.9.5 Ecological aspects of sulfide oxidation 389 8.08.8 COUPLED ANAEROBIC ELEMENT CYCLES 389 8.08.8.1 Evidence of Competitive Interactions 389 8.08.8.2 Mechanisms of Competition 390 &0&&3 ExcgfAofu 391 8.08.8.4 Noncompetitive Interactions 391 8.08.8.5 Contributions to Carbon Metabolism 391 8.08.8.6 Concluding Remarks 392 ACKNOWLEDGMENTS 392 REFERENCES 392 Overview of Life in the Absence of O2 319 8.08.1 OVERVIEW OF LIFE IN (Schlesinger, 1997). Anaerobic microorganisms THE ABSENCE OF 02 are common symbionts of termites, cattle, and many other animals, where they aid digestion. 8.08.1.1 Introduction Nutrient and pollutant chemistry are strongly Life evolved and flourished in the absence of modified by the reduced conditions that prevail in wetland and aquatic ecosystems. molecular oxygen (02). As the 02 content of the atmosphere rose to the present level of 21% This review of anaerobic metabolism empha- beginning about two billion years ago, anaerobic sizes aerobic oxidation, because the two pro- metabolism was gradually supplanted by aerobic cesses cannot be separated in a complete metabolism. Anaerobic environments have per- treatment of the topic. It is process oriented and sisted on Earth despite the transformation to an highlights the fascinating microorganisms that oxidized state because of the combined influence mediate anaerobic biogeochemistry. We begin of water and organic matter. Molecular oxygen this review with a brief discussion of C02 diffuses about 104 times more slowly through assimilation by autotrophs, the source of most water than air, and organic matter supports a large of the reducing power on Earth, and then consider the biological processes that harness biotic 02 demand that consumes the supply faster than it is replaced by diffusion. Such conditions this potential energy. Energy liberation begins exist in wetlands, rivers, estuaries, coastal marine with the decomposition of organic macromol- sediments, aquifers, anoxic water columns, sew- ecules to relatively simple compounds, which are age digesters, landfills, the intestinal tracts of simplified further by fermentation. Methanogen- animals, and the rumen of herbivores. Anaerobic esis is considered next because CH4 is a product microsites are also embedded in oxic environ- of acetate fermentation, and thus completes the ments such as upland soils and marine water catabolism of organic matter, particularly in the columns. Appreciable rates of aerobic respiration absence of inorganic electron acceptors. Finally, are restricted to areas that are in direct contact the organisms that use nitrogen, manganese, iron, with air or those inhabited by organisms that and sulfur for terminal electron acceptors are considered in order of decreasing free-energy produce 02. yield of the reactions. Rising atmospheric 02 reduced the global area of anaerobic habitat, but enhanced the overall rate of anaerobic metabolism (at least on an area basis) by increasing the supply of electron donors and 8.08.1.2 Overview of Anaerobic Metabolism acceptors. Organic carbon production increased dramatically, as did oxidized forms of nitrogen, Microorganisms derive energy by transferring manganese, iron, sulfur, and many other elements. electrons from an external electron source or In contemporary anaerobic ecosystems, nearly all donor to an external electron sink or terminal of the reducing power is derived from photosyn- electron acceptor. Organic electron donors vary thesis, and most of it eventually returns to 02, the from monomers that support fermentation to most electronegative electron acceptor that is simple compounds such as acetate and CH4. The abundant. This photosynthetically driven redox common inorganic electron donors are molecular gradient has been thoroughly exploited by aerobic hydrogen (H2), ammonium (NK|~), manganous and anaerobic microorganisms for metabolism. manganese (Mn(II)), ferrous iron (Fe(II)), and The same is true of hydrothermal vents hydrogen sulfide (H2S). Energy is harnessed by (Tunnicliffe, 1992) and some deep subsurface shuttling electrons through transport chains within environments (Chapelle et ah, 2002), where a cell until a final transfer is made to a terminal thermal energy is the ultimate source of the electron acceptor. The common terminal electron reducing power. acceptors are nitrate (NOJ), manganic manganese Although anaerobic habitats are currently a Mn(IV), ferric iron (Fe(III)), sulfate (SO4"), and small fraction of Earth's surface area, they have a carbon dioxide (C02) (Table 1). profound influence on the biogeochemistry of the Anaerobic organisms often have the capacity to planet. This is evident from the observation that reduce two or more terminal electron acceptors. In the 02 and CH4 content of Earth's atmosphere are many cases, these alternative reactions do not in extreme disequilibrium (Sagan et ah, 1993). support growth, as with the fermenting bacteria The combination of high aerobic primary pro- that reduce Fe(III) (Lovley, 2000b).
Details
-
File Typepdf
-
Upload Time-
-
Content LanguagesEnglish
-
Upload UserAnonymous/Not logged-in
-
File Pages108 Page
-
File Size-