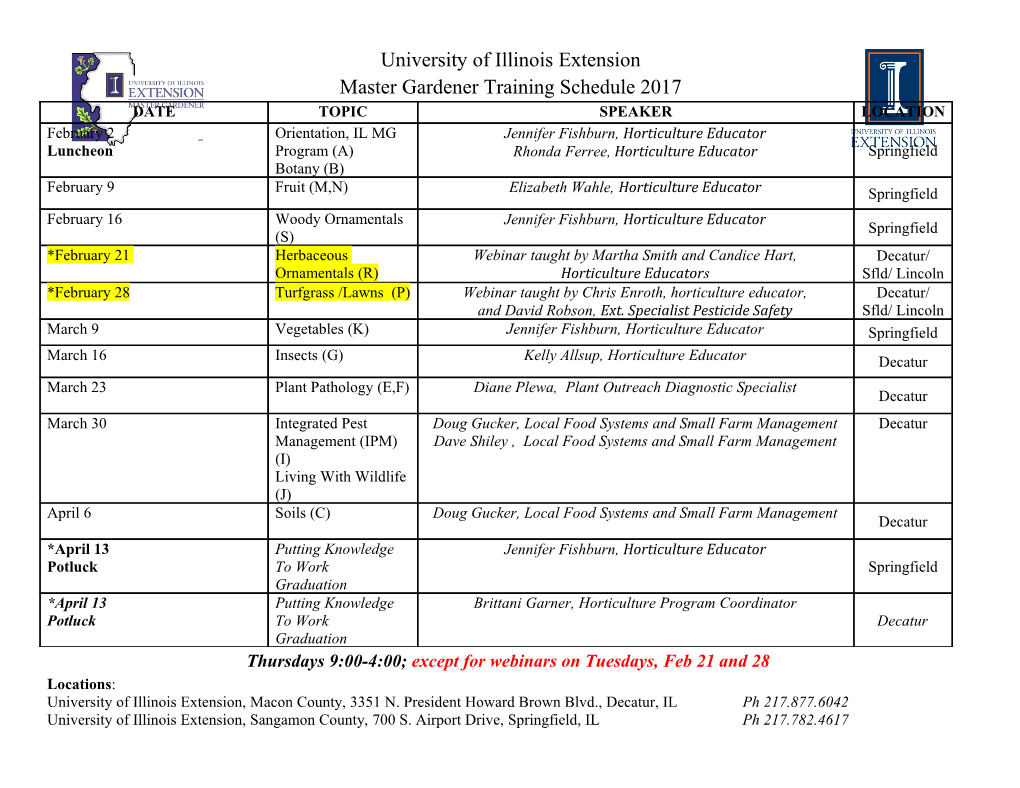
Chapter 4 Climate Change Mitigation with Renewable Energy: Geothermal Alper Baba Abstract On a global scale, there is increasing evidence that climate is changing and of a discernible human influence. Many of scientists are confident that if current emissions of greenhouse gases continue, the world will be warmer, sea levels will rise and regional climate patterns will change. According to some scientist, global temperatures are expected to rise faster over the next century than over any time during the last 10,000 years. From this token, geothermal energy is now considered to be one of the most important alternative energy sources to minimize climate change. Geothermal technologies for power generation or direct use operate with little or no greenhouse gas emissions. Geothermal energy is generally accepted as being an envi- ronmentally-friendly energy source, particularly when compared to fossil fuel energy sources. Geothermal resources have long been used for direct heat extraction for dis- trict urban heating, industrial processing, domestic water and space heating, leisure and balneotherapy applications. Geothermal energy is used in more than 80 countries for direct heat application and 24 countries for power generation. Re-injection of fluids maintains a constant pressure in the reservoir, thus increasing the field’s life and reducing concerns about environmental impacts. Geothermal energy has several significant characteristics that make it suitable for climate change mitigation. Keywords Climate change • Environment • Geothermal energy • Renewable energy 4.1 Introduction Due to global warming, global temperatures are expected to rise faster over the next century than any 100 years period during the past 10000 years [1–2]. Recent reports from the Intergovernmental Panel on Climate Change [3–4] confirm that climate A. Baba (*) Geothermal Energy Research and Application Center, Izmir Institute of Technology, Urla 35430, Izmir, Turkey e-mail: [email protected] A. Baba et al. (eds.), Climate Change and its Effects on Water Resources, 25 NATO Science for Peace and Security Series C: Environmental Security 3, DOI 10.1007/978-94-007-1143-3_4, © Springer Science+Business Media B.V. 2011 26 A. Baba change is occurring at a larger and more rapid rate of change than was thought likely only 6 years ago. Eleven of the last 12 years (1995–2006) rank among the 12 warm- est years in the instrumental record of global surface temperature (since 1850). The 100-year linear trend (1906–2005) of 0.74°C [0.56–0.92°C] is larger than the cor- responding trend of 0.6°C [0.4–0.8°C] (1901–2000) [1, 2, 5]. Climate warming observed over the past several decades is consistently associated with changes in a number of components of the hydrological cycle and hydrological systems such as: changing precipitation patterns, intensity and extremes; widespread melting of snow and ice; increasing atmospheric water vapour; increasing evaporation; and changes in soil moisture and runoff. There is significant natural variability in all components of the hydrological cycle, often masking long-term trends. There is still substantial uncertainty in trends of hydrological variables because of large regional differences, and because of limitations in the spatial and temporal coverage of monitoring net- works [6]. At present, documenting inter-annual variations and trends in precipita- tion over the oceans remains a challenge [3]. Greenhouse gases are considered to be the foremost parameter influencing the climate change. Carbon dioxide (CO2) is the most important anthropogenic green- house gas with annual emissions growing by about 80% between 1970 and 2004 [4]. The long-term trend of declining CO2 emissions per unit of energy supplied reversed after 2000. Global atmospheric concentrations of CO2, methane (CH4) and nitrous oxide (N2O) have increased markedly as a result of human activities since 1750 and now far exceed pre-industrial values determined from ice cores spanning many thou- sands of years. During the past 50 years, the sum of solar and volcanic forcing would likely have produced cooling. Observed patterns of warming and their changes are simulated only by models that include anthropogenic forcing [5]. Global carbon dioxide (CO2) emissions from residential, commercial, and institutional buildings are projected to grow from 1.9 Gt C/year in 1990 to 1.9–2.9 Gt C/year in 2010, 1.9–3.3 Gt C/year in 2020, and 1.9–5.3 Gt C/year in 2050. It must also be noted that 75% of the 1990 emissions are attributed to energy production [3]. Controlling greenhouse gases emission and adapting human settlements to with- stand the extreme climatic conditions have become the most formidable challenges of our times. Geothermal energy development has thus great CO2 emission reduc- tion potential when substituting fossil sources of energy. Geothermal energy is one of the contributors to any future energy mix. The advantages of geothermal energy are numerous. It is an environmentally friendly and economically rewarding resource, which is still only marginally developed. Its two main utilization catego- ries power generation and direct use are already introduced in many countries around the globe [7]. Geothermal development estimates for 2050 indicate that CO2 emissions could be mitigated by 100s of Mt/year with power generation from geo- thermal resources and more than 300 Mt/year with direct use, most of which could be achieved by geothermal heat pumps [8]. Based on these fundamentals, the pur- pose of this study is to explain application of geothermal energy and its effect on climate change and to assess environmental impacts of the utilization of geothermal resources. 4 Climate Change and Geothermal Energy 27 4.2 Application of Geothermal Energy People have been using geothermal energy for bathing and washing of clothes since the dawn of civilization in many parts of the world. However, it was first in the twentieth century that geothermal energy was used on a large scale for direct heat extraction for district urban heating, industrial processing, domestic water and space heating, leisure, balneotherapy applications and electricity generation. Prince Piero Ginori Conti initiated electric power generation with geothermal steam at Larderello in 1913. The first large scale municipal district heating service started in Iceland in 1930 [9]. In 2010, geothermal resources have been identified in more than 80 countries and there are quantified records of geothermal utiliza- tion in more than 50 countries in world. The result shows that use of geothermal energy for both electrical generation and direct heat extraction is going to accelerate in the near future. 4.2.1 Direct-Use of Geothermal Energy Direct-use of geothermal energy is one of the oldest, most versatile and also the most common form of utilization of geothermal energy [10]. The early history of geothermal direct-use has been well documented for over 25 countries in the Stories from a Heat Earth – Our Geothermal Heritage [11] that documents geother- mal use for over 2,000 years [12]. As of 2009, direct utilization of geothermal energy worldwide is 50,583 MWt. The total annual energy use is 438,071 TJ (121,696 GWh). The five countries with the largest installed capacities are: USA, China, Sweden, Norway and Germany accounting for 60% of the world’s capacity, and the five countries with the largest annual energy use are: China, USA, Sweden, Turkey, and Japan, accounting for 55% of the world use. However, an examination of the data in terms of land area or population shows that the smaller countries dominate, especially the Nordic ones. The largest increase in geothermal installed capacity (MWt) over the past 5 years are: United Kingdom, Korea, Ireland, Spain and Netherlands; and the largest increase in annual energy use (TJ/year) over the past 5 years are: United Kingdom, Netherlands, Korea, Norway and Ireland. All of these increases are due to geother- mal heat pump installations [12]. During the last decade, a number of countries have encouraged individual house owner to install ground source heat pumps to heat their houses in the winter and cool them in the summer. Summary of various categories of direct use worldwide is given in Fig. 4.1. The result shows that geothermal heat pumps were increased exponentially during the last 10 years. Other uses also increased with a linear trend. Geothermal fluids contain certain minerals leached from the reservoir rock and variable quantities of gas, mainly carbon dioxide and a smaller amount of 28 A. Baba 40000 35000 Geothermal Heat Pumps Geothermal Heat Pumps 30000 Space Heating Greenhouse Heating 25000 t Aquaculture Pond Heating 20000 Agricultural Drying MW 15000 Industrial Uses Bathing and Swimming 10000 Cooling/Snow Melting 5000 Others 0 1995 2000 2005 2010 Fig. 4.1 Summary of the various categories of direct use worldwide, referred to period 1995–2010 [12] hydrogen sulphide. The gas composition and quantity depend on the geological conditions encountered in different fields. Virtually the entire mineral content of the fluid and some of the gases are reinjected back into the reservoir. Most non- condensable gases are released to the environment. Some plants remove H2S in a gas treatment process before releasing CO2 to the environment. At one plant in Kizildere, Turkey, the non-condensable gases are scrubbed of H2S, and CO2 are recovered to provide about 80% of CO2 used by the country’s soft drinks industry [13]. 4.2.2 Power Generation of Geothermal Energy Electricity is produced with geothermal steam in 24 countries spread over all over the world. The worldwide total installed capacity of geothermal power plants is given in Fig. 4.2. The present value of 10.7 GW is an important result. The expected target from hydrothermal resources of 70 GW for year 2050 is very ambitious, as can be seen from Fig. 4.3 [14].
Details
-
File Typepdf
-
Upload Time-
-
Content LanguagesEnglish
-
Upload UserAnonymous/Not logged-in
-
File Pages9 Page
-
File Size-