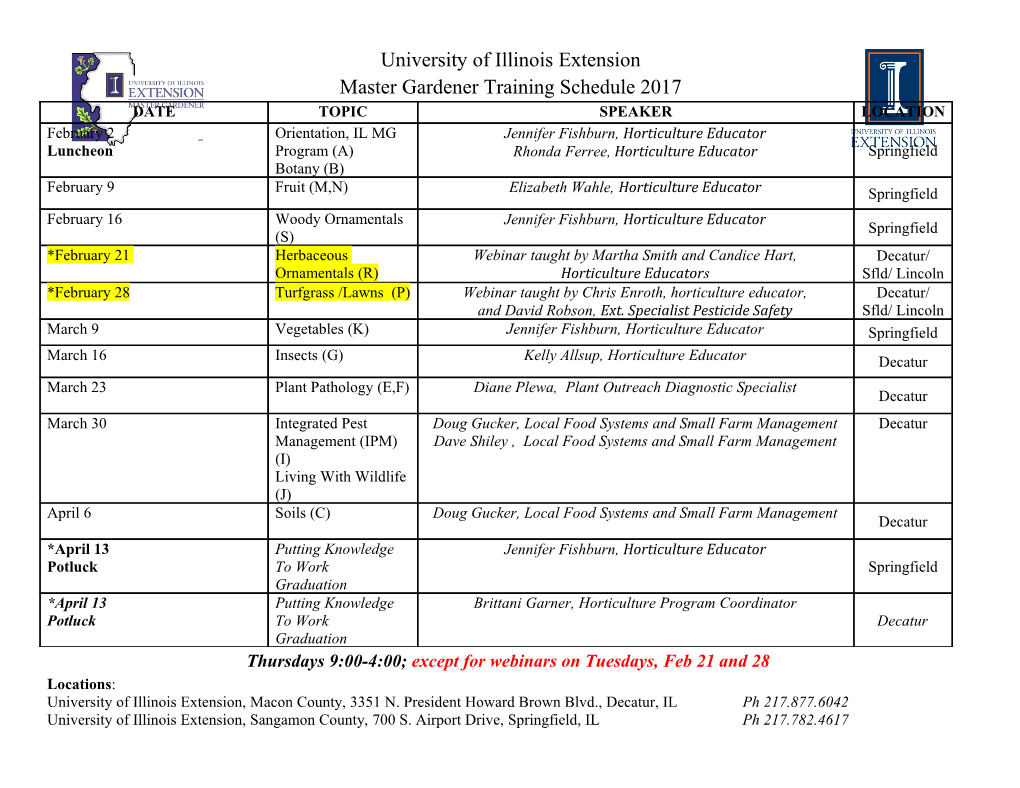
Fluorescence Introductory article Spectrophotometry Article Contents . The Electronic Excited State Peter TC So, Massachusetts Institute of Technology, Cambridge, Massachusetts, USA . Radiative and Nonradiative Decay Pathways Chen Y Dong, Massachusetts Institute of Technology, Cambridge, Massachusetts, USA . Factors Affecting Fluorescence Intensity . Phosphorescence . Fluorescence spectrophotometry is a class of techniques that assay the state of a biological Instrumentation for Fluorescence Spectrophotometry . Applications of Fluorescence in the Study of Biological system by studying its interactions with fluorescent probe molecules. This interaction is Structure and Function monitored by measuring the changes in the fluorescent probe optical properties. The Electronic Excited State indistinguishability of electrons and the Pauli exclusion Fluorescence and phosphorescence are photon emission principle require the electronic wave functions to have processes that occur during molecular relaxation from either symmetric or asymmetric spin states. The symmetric electronic excited states. These photonic processes involve wave functions, also called the triple state, have three transitions between electronic and vibrational states of forms, multiplicity of three. The antisymmetric wave polyatomic fluorescent molecules (fluorophores). The function, also called the singlet state, has one form, Jablonski diagram (Figure 1) offers a convenient represen- multiplicity of one. tation of the excited state structure and the relevant To the first order, optical transition couples states with transitions. Electronic states are typically separated by the same multiplicity. Optical transition excites the energies on the order of 10 000 cm 2 1. Each electronic state molecules from the lowest vibrational level of the electronic is split into multiple sublevels representing the vibrational ground state to an accessible vibrational level in an ele- modes of the molecule. The energies of the vibrational ctronic excited state. Since the ground electronic state is a levels are separated by about 100 cm 2 1. Photons with singlet state, the destination electronic state is also a singlet. energies in the ultraviolet to the blue-green region of the After excitation, the molecule is quickly relaxed to the spectrum are needed to trigger an electronic transition. lowest vibrational level of the excited electronic state. This Further, since the energy gap between the excited and rapid vibrational relaxation process occurs on the time ground electronic states is significantly larger than the scale of femtoseconds to picoseconds. This relaxation thermal energy, thermodynamics predicts that molecule process is responsible for the Stoke shift. The Stoke shift predominately reside in the electronic ground state. describes the observation that fluorescence photons are The electronic excited states of a polyatomic molecule longer in wavelength than the excitation radiation. can be further classified based on their multiplicity. The The fluorophore remains in the lowest vibrational level of the excited electronic state for a period on the order of nanoseconds, the fluorescence lifetime. Fluorescence emission occurs as the fluorophore decay from the singlet electronic excited states to an allowable vibrational level in S2 the electronic ground state. The fluorescence absorption and emission spectra reflect the vibrational level structures in the ground and the excited electronic states, respectively. The Frank–Condon principle states the fact that the vibrational levels are not Internal conversion significantly altered during electronic transitions. The E S1 Intersystem crossing similarity of the vibrational level structures in the ground and excited electronic states often results in the absorption and emission spectra having mirrored features. Excitation Fluoresence T1 The electronic excited state also has specific polarization properties. Fluorophores are preferentially excited when S0 the polarization of light is aligned along a specific Phosphorescence molecular axis (the excitation dipole). Further, the fluorescence photons subsequently emitted by the molecule Figure 1 The Jablonski diagram of fluorophore excitation, radiative decay and nonradiative decay pathways. E denotes the energy scale; S0 is the will have polarization orientated along another molecular ground singlet electronic state; S1 and S2 are the successively higher axis (the emission dipole). In general, the excitation and energy excited singlet electronic states. T1 is the lowest energy triplet state. emission dipoles do not coincide. ENCYCLOPEDIA OF LIFE SCIENCES / & 2002 Macmillan Publishers Ltd, Nature Publishing Group / www.els.net 1 Fluorescence Spectrophotometry Radiative and Nonradiative Decay External conversion describes the process where the fluorophore loses electronic energy to its environment Pathways through collision with other solutes. Collisional quenching processes are particularly interesting as they allow the Radiative decay describes molecular deexcitation pro- biochemical environment of the fluorophores to be cesses accompanied by photon emission. Molecules in the measured. A number of important solute molecules, such excited electronic states can also relax by nonradiative as oxygen, are efficient fluorescence quenchers. Upon processes where excitation energy is not converted into collision, the fluorophore is deexcited nonradiatively. The photons but are dissipated by thermal processes such as collisional quenching rate can be expressed as: vibrational relaxation and collisional quenching. Let G and k be the radiative and nonradiative decay rates respectively kec 5 k0[Q] [7] and N be the fraction of fluorophore in the excited state. The temporal evolution of the excited state can be where k0 is related to the diffusivity and the hydrodynamics described by: radii of the reactants and [Q] is the concentration of the quencher. dN When collisional quenching is the dominant nonradia- À À kN 1 dt tive process, eqn [1] predicts that fluorescence lifetime decreases with quencher concentration: ðÀþkÞt Àt=À N ¼ N0e ¼ N0e ½2 0 The fluorescence lifetime, t, of the fluorophore measures 1 k00Q 8 the combined rate of the radiative and nonradiative pathways: The steady state fluorescence intensity, F, also diminishes relative to the fluorescence intensity in the absence of 1 quencher, F0. This effect is described by the Stern–Volmer 3 À k equation: In the absence of nonradiative decay processes, one can F0 define the intrinsic lifetime of the fluorophore: 1 k00Q9 F 1 Fluorescence signal reduction can also result from ground 4 0 À state processes – steady state quenching. A fluorophore The ‘efficiency’ of the fluorophore can then be quantified can be chemically bound to a quencher to form a ‘dark by the fluorescence quantum yield, Q: complex’ – a product that does not fluoresce. Fluorescence intensity decreases with steady state quenching as: À Q 5 F0 À k 0 1 K Q10 F s where Ks is the association constant of the quencher and the fluorophore. Fluorescence lifetime is not affected by steady state quenching as the excited states are not Factors Affecting Fluorescence Intensity involved. A number of factors contributes to the nonradiative decay pathways of the fluorophores and reduces fluorescence intensity. In general, the nonradiative decay processes can Phosphorescence be classified as: Intersystem crossing is another process where fluorescence signal is reduced and phosphorescence is generated. Spin- k 5 kic 1 kec 1 kis [6] orbit coupling is a quantum mechanical process that is where kic is the rate of internal conversion, kec is the rate of responsible for intersystem crossing. Intersystem crossing external conversion, and kis is the rate of intersystem describes the relaxation of the molecule from a singlet crossing. excited state to a lower energy, triplet excitation state. Internal conversion is a process where the electronic Since spin-orbit coupling is a weak effect, the intersystem energy is converted to the vibrational energy of the crossing rate is low. The relaxation from the triplet state to fluorophore itself. Since vibrational processes are driven the singlet ground state requires another change of by thermal processes, the internal conversion rate typically multiplicity. Hence, the decay from the triplet states also increases with temperature, which accounts for the has a very low rate. However, radiative relaxation, commonly observed decrease in fluorescence intensity with phosphorescence, does occur due to spin-orbit coupling. rising temperature. The typical phosphorescence lifetime is on the order of 2 ENCYCLOPEDIA OF LIFE SCIENCES / & 2002 Macmillan Publishers Ltd, Nature Publishing Group / www.els.net Fluorescence Spectrophotometry microseconds to seconds. Phosphorescence has larger EXO SC Stoke shift than fluorescence owing to the triple state having lower energy. LS Since phosphorescence rate is often much lower than thermally activated nonradiative decay processes such as collisional quenching, phosphorescence is rarely observed in aqueous systems at physiological temperature. How- ever, a number of protein conformation studies at cryogenic temperatures have utilized phosphorescence spectroscopy. EMO Instrumentation for Fluorescence Spectrophotometry The measurement of fluorescence signals provides a DET sensitive method of monitoring the biochemical environ- ment of a fluorophore. Instruments have been designed to Figure 2 A typical fluorometer design. LS is the light source, EXO is the measure
Details
-
File Typepdf
-
Upload Time-
-
Content LanguagesEnglish
-
Upload UserAnonymous/Not logged-in
-
File Pages4 Page
-
File Size-