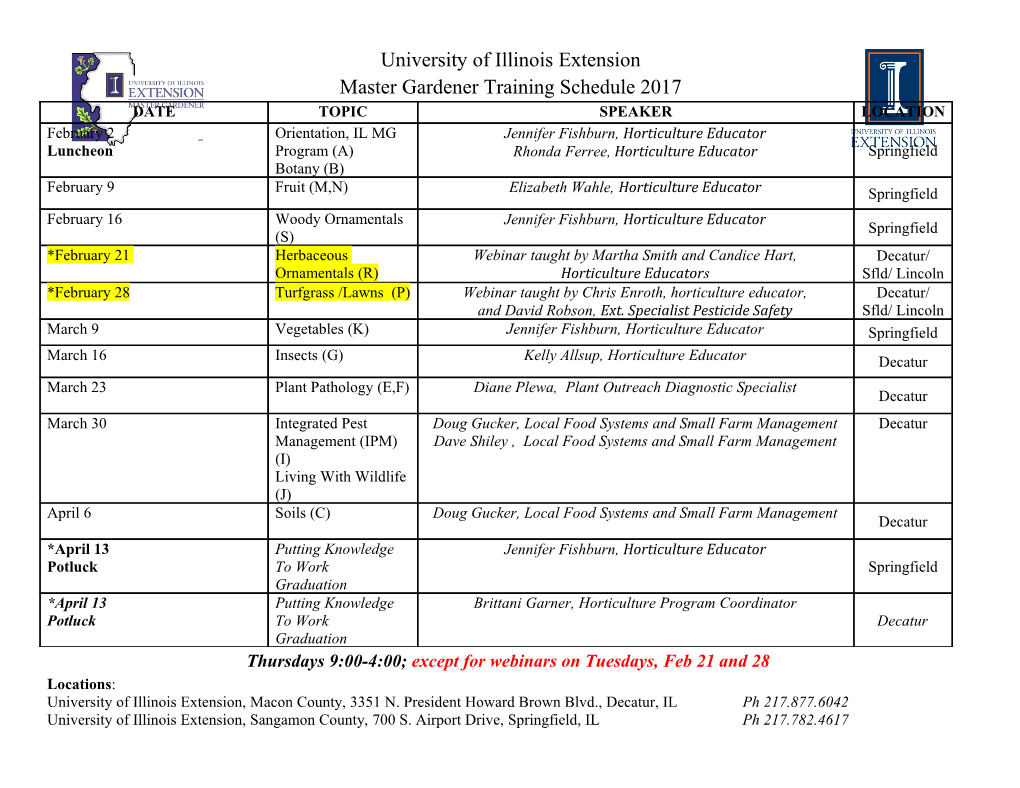
Appendix A Standard Distributions A.1 Standard Univariate Discrete Distributions I. Binomial Distribution B(n, p) has the probability mass function n f(x)= px (x =0, 1,...,n). x The mean is np and variance np(1 − p). II. The Negative Binomial Distribution NB(r, p) arises as the distribution of X ≡{number of failures until the r-th success} in independent trials each with probability of success p. Thus its probability mass function is r + x − 1 r x fr(x)= p (1 − p) (x =0, 1, ··· , ···). x Let Xi denote the number of failures between the (i − 1)-th and i-th successes (i =2, 3,...,r), and let X1 be the number of failures before the first success. Then X1,X2,...,Xr and r independent random variables each having the distribution NB(1,p) with probability mass function x f1(x)=p(1 − p) (x =0, 1, 2,...). Also, X = X1 + X2 + ···+ Xr. Hence ∞ ∞ x x−1 E(X)=rEX1 = r p x(1 − p) = rp(1 − p) x(1 − p) x=0 x=1 ∞ ∞ d d = rp(1 − p) − (1 − p)x = −rp(1 − p) (1 − p)x x=1 dp dp x=1 © Springer-Verlag New York 2016 343 R. Bhattacharya et al., A Course in Mathematical Statistics and Large Sample Theory, Springer Texts in Statistics, DOI 10.1007/978-1-4939-4032-5 344 A Standard Distributions ∞ d d 1 = −rp(1 − p) (1 − p)x = −rp(1 − p) dp dp 1 − (1 − p) x=0 =p r(1 − p) = . (A.1) p Also, one may calculate var(X) using (Exercise A.1) var(X)=rvar(X1). (A.2) III. The Poisson Distribution P(λ) has probability mass function λx f(x)=e−λ (x =0, 1, 2,...), x! where λ>0 is the mean. To see this let X be a random variable with this distribution. Then ∞ x ∞ x ∞ y −λ λ −λ λ −λ λ E(X)= xe = e − = λe x=0 x! x=1 (x 1)! y=0 y! = λe−λ · eλ = λ. (y = x − 1) Also, ∞ ∞ λx λx E(X(X − 1)) = x(x − 1)e−λ = e−λ x(x − 1) x=0 x! x=2 x! ∞ x−2 −λ 2 λ −λ 2 · λ 2 = e λ − = e λ e = λ , x=2 (x 2)! so that E(X2)=λ2 + E(X)=λ2 + λ, var(X)=E(X2) − (E(X))2 = λ2 + λ − λ2 = λ. (A.3) IV. The Beta-Binomial Distribution BB(α, β, n) is the marginal distribution of X when the conditional distribution of X given (another random variable) Y = y (withvaluesin[0, 1]) is B(n, y), where Y has the beta distribution Be(α, β)(see Sect. A.2). Hence the probability mass function of X is 1 n 1 f(x)= yx(1 − y)n−x yα−1(1 − y)β−1dy x B(α, β) 0 n n 1 B(x + α, n − x + β) = x yx+α−1(1 − y)n−x+β−1dy = x B(α, β) 0 B(α, β) n Γ (x + α)Γ (n − x + β)Γ (α + β) = (x =0, 1,...,n) x Γ (n + α + β)Γ (α)Γ (β) A Standard Distributions 345 [See below for the relation B(α, β)=Γ (α)Γ (β)/Γ (α + β).]HereifX ∼ BB(α, β, n), nα E(X)=EE(X | Y )=EnY = nEY = , α + β E(X2)=EE(X2 | Y )=E[nY (1 − Y )+n2Y 2] α(α +1) α =(n2 − 1)EY 2 + nEY =(n2 − 1) + n , (α + β)(α + β +1) α + β var (X)=E(X2) − (E(X))2. (See below for the computation of the moments of the beta distribution.) A.2 Some Absolutely Continuous Distributions I. The Uniform Distribution U (α, β)on[α, β] has the probability density function (p.d.f.) 1 f(x)= for α ≤ x ≤ β, β − α = 0 elsewhere. II. The Beta Distribution Be(α, β) has p.d.f. 1 f(x)= xα−1(1 − x)β−1, 0 <x<1, B(α, β) = 0 elsewhere. Here α>0, β>0andB(α, β) is the normalizing constant (beta function) 1 B(α, β)= xα−1(1 − x)β−1dx. 0 Clearly, if X ∼ Be(α, β), then 1 1 B(α +1,β) E(X)= xα(1 − x)β−1dx = , B(α, β) 0 B(α, β) B(α +2,β) B(α + k, β) E(X2)= ,..., E(Xk)= . (A.4) B(α, β) B(α, β) Recall that the gamma function Γ (α) is defined by ∞ Gamma(α)= e−xxα−1dx (α>0). 0 On integration by parts one gets ∞ ∞ ∞ Γ (α +1)= e−xxαdx = −e−xxα + αxα−1e−xdx =0+αΓ (α)=αΓ (α). 0 0 0 (A.5) 346 A Standard Distributions We now prove Γ (α)Γ (β) B(α, β)= , ∀ α>0,β>0. (A.6) Γ (α + β) Now ∞ ∞ Γ (α)Γ (β)= e−xxα−1dx e−yyβ−1dy 0 0 ∞ ∞ = e−(x+y)xα−1yβ−1dxdy. 0 0 x = z − y Change variables: z = x + y, y = y,toget , with y = y ∂x ∂x ∂t ∂y Jacobian = det ∂y ∂y =1 ∂z ∂y ∞ z Γ (α)Γ (β)= e−z (z − y)α−1yβ−1dy dz 0 0 ∞ z y α−1 y β−1 = e−zzα−1zβ−1 1 − dy dz z z 0 0 ∞ 1 −z α+β−2 α−1 β−1 y 1 = e z z (1 − u) u du dz u = z ,du= z dy 0 0 ∞ = e−zzα+β−1B(β,α)dz = Γ (α + β)B(β,α). (A.7) 0 But 1 1 B(β,α)= uβ−1(1 − u)α−1du = xα−1(1 − x)β−1dx 0 0 = B(α, β), (x =1− u). (A.8) Hence (A.7)and(A.6). Using (A.4)–(A.6), one gets the k-th moment of a beta (Be(α, β)) random variable X as Γ (α + k)Γ (β) · Γ (α + β) (α + k − 1) ···(α +1)α E(Xk)= = . Γ (α + β + k) · Γ (α)Γ (β) (α + β + k − 1) ···(α + β +1)(α + β) In particular, α (α +1)α αβ E(X)= ,E(X2)= , var(X)= . α+β (α + β +1)(α + β) (α+β)2(α+β+1) III. The Gamma Distribution G (α, β) has the p.d.f. 1 f(x)= e−x/αxβ−1, 0 <x<∞ Γ (β)αβ = 0 elsewhere, (A.9) A Standard Distributions 347 where α>0, β>0. Here α is a scale parameter, i.e., if X is ∼ G (α, β), then X/α is G (1,β), Note that k X 1 ∞ E = zke−zzβ−1dz α Γ (β) 0 Γ (β + k) = =(β + k − 1) ···(β +1)β, Γ (β) so that E(Xk)=αk(β + k − 1) ···(β +1)β. (A.10) Hence EX = αβ,var(X)=α2β. A.2.1 The Normal Distribution N(μ, σ2) has p.d.f. 1 −(x−μ)2/2σ2 fμ,σ2 (x)=√ e , −∞ <x<∞, (A.11) 2πσ2 where μ ∈ (−∞, ∞), σ2 > 0. The standard normal distribution N(0, 1) has p.d.f. 1 2 f(x)=√ e−x /2, −∞ <x<∞. (A.12) 2π x−μ To show that (A.12) (and hence (A.11), by transformation y = σ ) is a p.d.f., one needs to show that ∞ 2 π e−x /2dx = (A.13) 0 2 For this use the transformation z = x2/2toget ∞ ∞ √ −x2/2 −z 1 − 1 1 1 e dx = e 2 z 2 dz = √ Γ . (A.14) 0 0 2 2 2 1 Now, by (A.7)(withα = β = 2 ) 1 1 1 1 1 Γ 2 = Γ (1)B , =B , (since Γ (1)= ∞ e−xdx= − e−x|∞ =1, ) 2 2 2 2 2 0 0 1 1 = x−1/2(1 − x)−1/2dx = z−1(1 − z2)−1/22zdz (z = x1/2,dx=2zdz) 0 0 1 π/2 cos θdθ =2 (1 − z2)−1/2dz =2 (z =sinθ, dz =cosθdθ) 0 0 cos θ π =2 = π. 2 Hence 1 √ Γ = π, (A.15) 2 whichwhenusedin(A.14) yields (A.13). 348 A Standard Distributions 2 X−μ If X is N(μ, σ ), then Z = σ has the p.d.f. (A.12) (by change of variables). Therefore, ∞ X − μ 1 2 2 E = E(Z)= √ xe−x /2dx =0 (sincexe−x /2 is odd ), σ 2π −∞ 2 ∞ ∞ X − μ 1 2 2 2 E = E(Z2)=√ x2e−x /2dx = √ x2e−x /2dx σ 2π 2π −∞ 0 ∞ 2 1 −z 2 = (2z) 2 e dz (z = x ,dz= xdx) π 2 0 2 ∞ 2 3 2 1 1 2 1 √ = √ e−zz1/2dz = √ Γ = √ Γ = √ π π 0 π 2 π 2 2 π 2 =1. Hence E(X − μ)=0, or E(X)=μ, 2 X−μ 2 (A.16) E σ =1, or, var(X)=σ . 2 V. The Chi-Square Distribution χk with k Degrees of Freedom is defined to be the distribution of the sum of squares of k independent standard normal random variables. To derive its p.d.f. let X1,X2,...,Xk be k independent N(0, 1) random variables. Then define the chi-square random variable 2 2 2 Z = X1 + X2 + ···+ Xk , and note that, as Δz ↓ 0, k 1 −(x2+···+x2 )/2 P (z<Z≤ z + Δz)= ··· √ e 1 k dx1 ···dxk 2π k 2 {(x1,...,xk):z< xi ≤ z + Δz} 1 k 1 = √ e−z/2 + O(Δz) 2π 2 ×volume of the annulus {(x1,...,xk):z< xi <z+ Δz} k √ k √ 1 −z/2 k = √ e + O(Δz) ck z + Δz − ck z , 2π k writing the volume of a ball of radius r in k-dimension as ckr .
Details
-
File Typepdf
-
Upload Time-
-
Content LanguagesEnglish
-
Upload UserAnonymous/Not logged-in
-
File Pages45 Page
-
File Size-