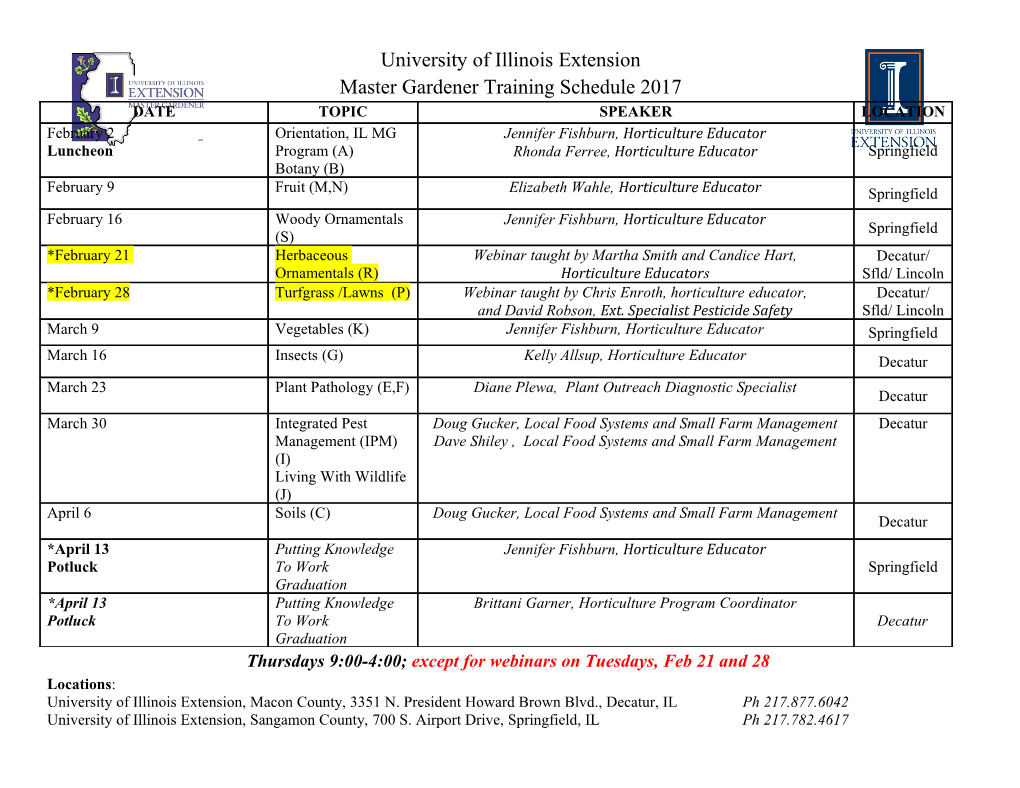
See discussions, stats, and author profiles for this publication at: https://www.researchgate.net/publication/305467432 Non-vacuum silvering and over-coating of telescope mirrors Article · January 2011 READS 17 5 authors, including: Lisa Brodhacker Lander University 9 PUBLICATIONS 75 CITATIONS SEE PROFILE All in-text references underlined in blue are linked to publications on ResearchGate, Available from: Lisa Brodhacker letting you access and read them immediately. Retrieved on: 15 August 2016 Non-Vacuum Silvering and Overcoating of Telescope Mirrors K. Lisa Brodhacker, Genevieve Harris, Bruce Holenstein, Sagar Venkateswaran, and Russell Genet Introduction For over a century, astronomical mirrors were made reflective via the fairly simple, low cost chemical deposition process of silvering. This process did not require an expensive vacuum chamber and could be accomplished by amateur telescope makers (ATMs) in their home shops. Although some amateur astronomers still silver their mirrors—especially in Europe—most ATMs who make their own mirrors now ship them off to have them vacuum aluminized and overcoated. Vacuum coating, which typically consists of a thin aluminum reflective layer and a clear, hard, transparent overcoat, has two distinct advantages. First, the reflective layer does not tarnish over time as unprotected silver does and, second, the transparent overcoat protects the delicate reflective layer from environmental degradation or being scratched while cleaning mirrors. Although the vacuum aluminizing and overcoating process is now well established for astronomical mirrors, it does have drawbacks in some situations. While the process is not expensive for small mirrors, it rapidly becomes more expensive as mirrors get larger. Large vacuum chambers are expensive to build, maintain, and operate. Furthermore, it is expensive to ship large mirrors to vacuum coaters, and it does expose the mirrors to shipping hazards. Finally, some of the more interesting low cost, lightweight mirrors currently being developed are made from materials other than glass, such as spin-cast epoxy mirrors. Due to outgassing of non-glass materials, chamber owners are reluctant to place such mirrors in their chambers for fear of chamber contamination. Since the cost of vacuum aluminizing and overcoating appears that it might actually exceed the cost of the large, lightweight, low cost mirrors, we decided it was time to reconsider the chemical deposition of silvering. If some low cost, easily applied process could be developed to overcoat silvered mirrors with a tough, transparent layer without recourse to a vacuum chamber, then the advantages of silvering could be retained without its disadvantages. We would not expect a newly developed, non-vacuum silvering/overcoating process to replace vacuum aluminizing/overcoating in most situations. However, it could be advantageous for “large” meter-class situations where low cost was vital, ease of application in a “home shop” environment was desired, or mirrors were made from exotic (outgassing) materials that would not be allowed in a vacuum chamber. Silvering The mirror in Newton’s first 1.3-inch reflecting telescope in 1668 was made from speculum (Latin for mirror), a metal alloy of roughly two-thirds copper and one-third tin—often with a touch of arsenic. Because speculum tarnished rapidly, the mirrors had to be re-polished and refigured at frequent intervals. This process was facilitated by having at least two mirrors for each telescope; one was refigured while the other was used to observe. These were not multiple mirror telescopes in the modern sense of the term! It wasn’t until 1856, almost 200 years later, that Karl von Steinheil and Leon Foucault were able to chemically deposit a very thin layer of silver on glass to create first-surface astronomical mirrors. When the silver tarnished, it could be removed chemically and a new silver layer deposited without having to re-polish and refigure the mirror. Thus only one mirror was required per telescope. Silvering produced high quality optical mirror surfaces for many years, and the process was refined and simplified in the 1930’s when William Peacock (the founder of Peacock Laboratories) invented a spray process that combined the reactants at the last second as they came out of twin nozzles and were sprayed onto the mirrors. Recently, Peacock Laboratories has developed new formulations under the PChrome brand to meet market demands for brighter first-surface silver coatings on both 1 transparent and nontransparent substrates. This new Peacock Laboratories silvering process involves four steps. 1. Degreasing: apply a 3% solution (all dilutions using deionized water unless explicitly stated) of #77 cleaner on the as-received polished glass samples, followed by deionized water rinsing. 2. Sensitization: apply, using a sensitizer spray gun, a 3% PChrome D solution, followed by DI water rinsing. 3.Silvering: using a silver spray double nozzle gun, spray 3% solution of PChrome S silver solution and a 3% solution of PChrome RX reducer solution until a bright, highly reflective first surface mirror is obtained. This is followed by DI water rinsing and drying the mirror completely before the final step of overcoating. 4. Overcoating: Permalac 2KA, Permalac 2K B and #281 thinner are mixed 1:1:1 and sprayed with a paint spray gun on the mirrored surfaces and allowed to dry overnight before optical testing. Non-Vacuum Overcoating As suggested above, non-vacuum silvering via chemical deposition and astronomical mirrors went together for over a century. However, once vacuum aluminizing and overcoating became available, recoating tarnished silvered mirrors became a burden. Furthermore, the bare silver coatings were not scratch resistant, making mirror cleaning a delicate job. If the problem of tarnishing could be solved by some non-vacuum chemical deposition problem, then perhaps mirrors could be silvered and overcoated at very low cost without having to be shipped to a distant vacuum coater (or being turned down for having an exotic mirror material). We accepted the challenge to review and try some chemical processes, such as sol-gel with nano-particles, to see if a suitable transparent overcoating material could be found for silvered mirrors. We realize, of course, that while the final optical quality of a chemical deposition overcoating process probably will not match that of a multi-layer vacuum overcoat, there are still a number of astronomical applications where gathering large numbers of photons at low cost is appropriate, and the optical quality of the coating is not critical. One such application is on-axis flux collectors, i.e. light buckets (Genet and Holenstein 2010). Sol-Gel Process The sol-gel process has been used since the middle 1800’s to produce ceramic and glass materials. The chemistry and physics have been studied extensively since the 1930’s and a renewed interest arose in the 1970’s due to the ease of the process (Hench 1990). The sol-gel technique is now routinely used in the field of material science as well as ceramic production because the properties of the final polymer can be tuned accordingly. Thin films, optical fibers, ultra-fine powders, and optical lenses are just a few of the numerous products derived from this process. The most common precursors in the sol-gel process are silicon or other metal alkoxides because they react readily with water. As shown below, the alkoxide groups are replaced with hydroxyl groups which then condense, leading to the removal of a water molecule. The strength of the resulting polymer is dependent on the functionality of the starting material (mono-, di-, tri- or tetra-functional). 2 H3CO HO Si + 2 H2O Si + 2 HOCH3 OCH3 OH An example of a di-functional silicon alkoxide HO OH HO OH Si + Si Si Si + H2O OH HO O Reaction occurs at every OH group The chemistry of a silicon alkoxide in the sol-gel process. Sol Gelation Agglomeration Gel Aging and Drying The sol-gel process includes the preparation of small nanoparticles (the sol), agglomeration of those nanoparticles (the gel), and further chemical reactions, leading to a dense polymer network. There are four basic stages for the sol-gel process as shown in Hench (1998): First Stage A stable solution of dispersed nanoparticles (the sol) is formed through hydrolysis or condensation reactions. The size of the nanoparticles can be controlled by varying the pH, temperature, length of reaction, use of catalysts, and varying the functionality of starting materials. Since this stage produces a very low-viscosity liquid, it is often added to a mold to produce monolithic ceramics or glasses. Second Stage Once the nanoparticles are formed, they will begin to agglomerate and eventually form a growing network (the gel). The point at which the viscous liquid changes to an elastic gel is 3 known as the gel point. This gelation, which is marked by a sudden and irreversible rise in viscosity, marks the point where a network of near infinite molecular weight first appears. Just as with the sol, the physical properties of the polymer can be controlled and are dependent upon the size of the nanoparticles, pH, functionality of starting material, and time to gelation. Third Stage Usually following gelation is another transformation called vitrification. Vitrification is the point where the gel changes to a glassy solid and is associated with an increase in crosslink density. This is the “aging stage” where as the hydrolysis or condensation reactions continue, a solid mass emerges. This is accompanied by a spontaneous shrinkage, which may result in cracks in the gel. The best way to prevent this is to allow the aging to occur over a long period of time. Fourth Stage During the drying stage, water and solvents are removed from the gel network at temperatures below 200°C. The drying must occur slowly so the aged gel can gain strength and resist cracking. Other stages include high temperature stabilization and densification to lead to ceramics.
Details
-
File Typepdf
-
Upload Time-
-
Content LanguagesEnglish
-
Upload UserAnonymous/Not logged-in
-
File Pages7 Page
-
File Size-