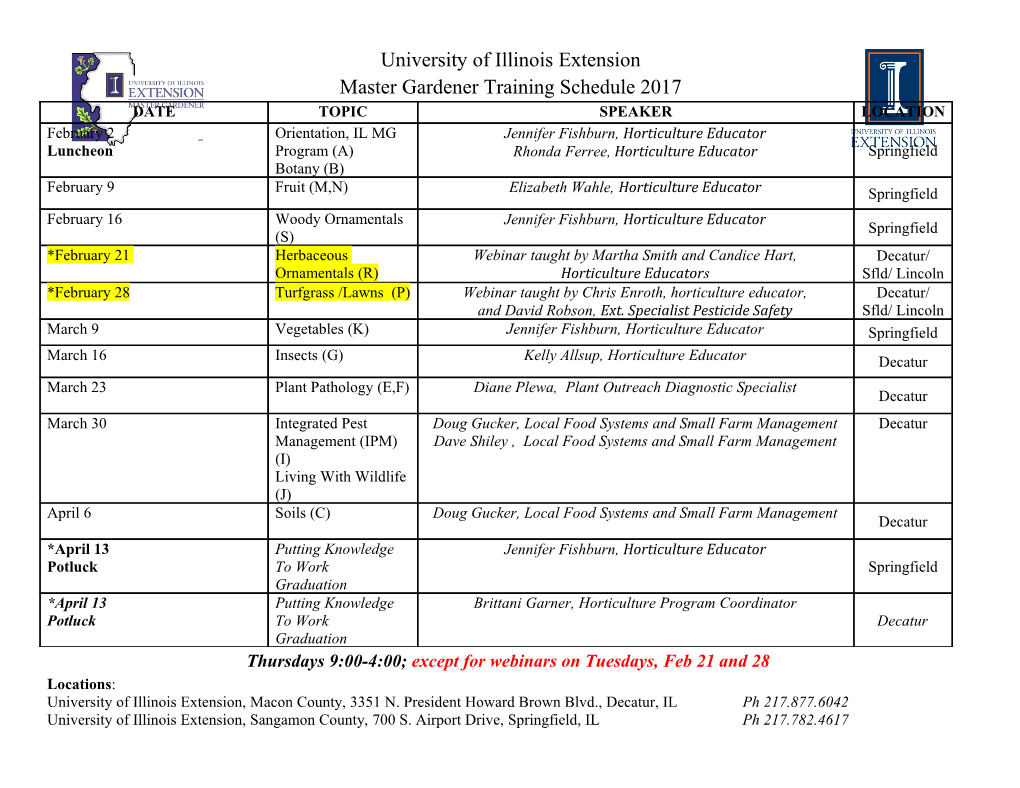
Characterization of nanoparticles for brain cancer treatment using magneto plasmonic approach Tina Seyedjamali1† , Mohamadreza Kazem Farahzadi2, and Hossein Arabi3 1Department of Physics, Iran University of Science and Technology, Narmak, Tehran 16846, Iran 2Department of Radiation Medical Engineering, Science and Research Branch, Islamic Azad University, Tehran, Iran 3Division of Nuclear Medicine and Molecular Imaging, Department of Medical Imaging, Geneva University Hospital, CH-1211 Geneva 4, Switzerland ABSTRACT This study investigates the treatment of brain cancer by the magnetic hyperthermia approach and nanoparticles including 퐹푒푂 core with gold, silver alloy shell, and 푀표푆 coating. Optical properties of these nanoparticles within the tumor, including the extinction coefficient and surface plasmon peak (SPR) as a function of size, structure, different compositions, and thickness are also investigated using the effective medium theory. Moreover, the impact of temperature distribution was assessed through the analytical modeling of alternating current (AC) magnetic field. The results of this study indicated that nanoparticles with a compound of 퐹푒푂 − 퐴푢.퐴푔.@푀표푆 and a thickness of 3 nm of gold-silver alloy and 3 layers of 푀표푆 have the best coefficient of extinction and SPR in the biological window. The gold-silver alloy improved the extinction coefficient and, at the same time, prevented the accumulation of magnetic nanoparticles. Since the gold-silver alloy alone cannot function within the range of biological windows, 푀표푆 was used, which increased the extinction efficiency at higher wavelengths. Examination of the temperature distribution in the tumor for the proposed alloy compound indicated that after a short time from the start of irradiation, the tumor temperature reaches 45 ° C. Also, the temperature distribution within the tumor tissue reached its maximum value at the center of the tumor and decreased dramatically as getting away from the center. The use of magnetic hyperthermia enabled localized delivery of therapeutic dose to malignant brain tumors; hence, exhibiting superior performance/efficiency over the photothermal method. KEYWORDS: Magnetic Hyperthermia Treatment (MHT), Brain cancer, Magnetic Nanoparticles, Surface Plasmon Resonance (SPR), Extinction coefficient 1. INTRODUCTION Since cancer cells are more sensitive to temperature, recent therapeutic initiatives have mostly relied on the heating characteristics of the tumor cells. Although the heating techniques alone are not sufficient, they could effectively destroy cancerous tissues, and hence can be considered as a complementary treatment [1, 2]. Hyperthermia is known as one of the minimally invasive therapies based on the conversion of energy sorces including microwaves, radio waves and ultrasound into heat. In this method, nanoparticles with high energy absorption and high light-to-heat conversion efficiency are used in the near-infrared (NIR) region [3]. In the hyperthermia technique, the temperature within the tumor commonly ranges from 41 to 45 ° C. S.K. Sharma et al. showed that when the temperature rises above 46 ° C, which is known as thermal erosion, it can effectively result in cell necrosis [4-6] . In the laser interstitial thermal therapy (LITT) technique, proposed by Skandalaki et al., surgery is required to create a field to place the cavity in the tissue, wherein the creation of cavities or invasive operations may adversely affect healthy tissues [7]. On the other hand, Yue He et al. have shown that photothermal therapy cannot treat deep tumors such as brain lesions, since the skull does not allow the emitted wavelength to pass through; and thus, the heat delivery cannot be effectively controlled [8]. To address this limitation, magnetic hyperthermia was proposed for deep lesions [9, 10]. In this technique, heat is generated by applying an alternating magnetic field to the nanoparticles within the target tissue [11]. The choice of nanoparticles depends on the ranges/levels of temperature, the efficiency of heat production, duration of treatment, toxicity, solubility in biofuels fluids, damage to the healthy tissue, and efficiency in destroying the cancerous tissue. The favorable nanoparticles should have high coefficients of extinction and adsorption in the range of biological windows (680- 1400 nm) in the target tissue [12-14]. Moreover, the delivery of heat to the target tissue would also be affected by the environment, size, geometry, and structure of nanoparticles [15, 16]. Heat generation properties of different types of nanoparticles have been studied for having efficient hyperthermia treatment. In this regard, bimetallic nanoparticles have favorable optical properties (better heat generation properties) than single metal nanoparticles [17]. Through modifying the nanostructure composition, the surface plasmon resonance (SPR) and the extinction coefficient can be adjusted within the desired window. Gold is biologically compatible as it is a noble metal, which is thermally and chemically stable [15, 18, 19]. Despite these good properties of gold for heat treatment, pure gold would result in a poor extinction rate. Silver nanoparticles are the other nanoparticle category which have been widely used in medical trials due to their antibiotic potential, but when they are utilized alone, the Ag + produced through oxidation could hinder the process of heat production [20, 21]. It should also be noted that silver alone is not biocompatible. Therefore, in recent years, the use of two-dimensional (very thin) materials, especially graphene and Transition Metal Dichalcogenides (TMDC) for heat treatment has received much attention [22]. Of the four commonly used TMDC (namely 푀표푆, 푊푆, 푀표푆푒, 푊푆푒), only 푀표푆 is biodegradable, and it is eliminated from the body within approximately one month [23, 24]. Wang et al. utilized graphene oxide to treat brain cancer in comparison with 푀표푆, wherein 푀표푆 nanomaterials exhibited about 7.8 times more energy absorption (i.e., graphene oxide led to a much higher coefficient of extinction than graphene). Moreover, 푀표푆 can be heated rapidly under NIR laser radiation. As a result, high-efficiency 푀표푆 nanomaterials have gained popularity owing to their effective response to NIR light radiation in cancer treatment [14, 23, 25]. Recently, magnetic nanoparticles have been considered as an important agent in hyperthermia. Employing these magnetic nanoparticles carriers would increase the penetration/absorption of drug in the cancer cell membrane. Hence, they can prevent systematic administration and side effects, and increase the therapeutic effect of anti-cancer drugs [26-29]. Iron oxide has been extensively studied because of its fascinating properties such as easy synthesizing process, low cost, biocompatibility, depth of penetration and most importantly, superparamagnetism, compared to other materia [30, 31]. Although iron oxide nanoparticles are biocompatible, they are usually coated with biocompatible polymers to prevent oxidation for hyperthermia treatment [32, 33]. The most common form of iron oxide is 퐹푒푂 (combination of divalent and trivalent iron oxides) [34]. In this paper, relying on effective medium theory, bio-heat and magnetic equations were employed to investigate the extinction coefficients and SPR as a function of core radius, the number of layers, environment effect, and the percentage of alloy compounds for 퐹푒푂 − 퐴푢 − 퐴푔@푀표푆 structure. 2. MATERIALS AND METHODS In this section, we examine the optical and thermal properties of the 퐹푒푂,퐴푢 − 퐴푔@푀표푆 structure Figure 1. First, we relied on the dedicated equations to model the effect of the number of layers on the optical properties of the nanoparticle, then we examined the different percentages of the alloy components and calculated the extinction efficiency and SPR. In the third section, regarding the magnetic nanoparticles, we examined the governing equations of magnetism; and finally, we investigated the bio-heat equations and temperature distribution. This study relied on the effective medium theory, which is based on the wighted average theory, Bruggeman's theory, and Maxwell Garnet's theory [35-38]. Figure 1: Schematic of nanoparticle with 퐹푒푂 core and 퐴푢 − 퐴푔@푀표푆 shell. 2.1 Effective medium theory for 푴풐푺ퟐ coated with Au-Ag In this section, we employed the governing equations for the number of layers using effective medium theory. Maxwell-Garnet's theory is based on the Clausius-Mossotti relation, which considers the correlation between polarization 훼 and the dielectric phenomenon [39]. In this regard, it is supposed that several layers of different nanoshells are stacked on top of each other [39]. As shown in Figure 1, there is an Au-Ag spherical nanoshell with an inner radius of 푟 and a thickness of 푡 surrounded by 푀표푆 with a thickness of 푡, while the core of this nanoparticle is of 퐹푒푂 (15,20 and 25 nm). The refractive indices of the healthy tissue and the tumor are 1.82 and 2.12, respectively. 휀(휔), 휀(휔) and 푖 = 1,2,…, denote the dielectric function of the core and the shell, respectively. The dielectric performance of the target external environment is frequency- dependent, which is equal to 휀(휔) [40]. According to Maxwell-Garnet’s theory, the dielectric effect between the core and the first shell is formulated as follows [40]: 휀(1 + 2푓) + 휀(2 − 2푓) (1) 휀, = 휀 휀(2 + 푓) + 휀(1 − 푓) Where the volume fraction of the new layer is obtained from: 푓 = (푟⁄푟) (2) and for other layers, Eq. (3) could be derived from Eqs. (2) and (3) 휀,(1 + 2푓) + 휀(2
Details
-
File Typepdf
-
Upload Time-
-
Content LanguagesEnglish
-
Upload UserAnonymous/Not logged-in
-
File Pages22 Page
-
File Size-