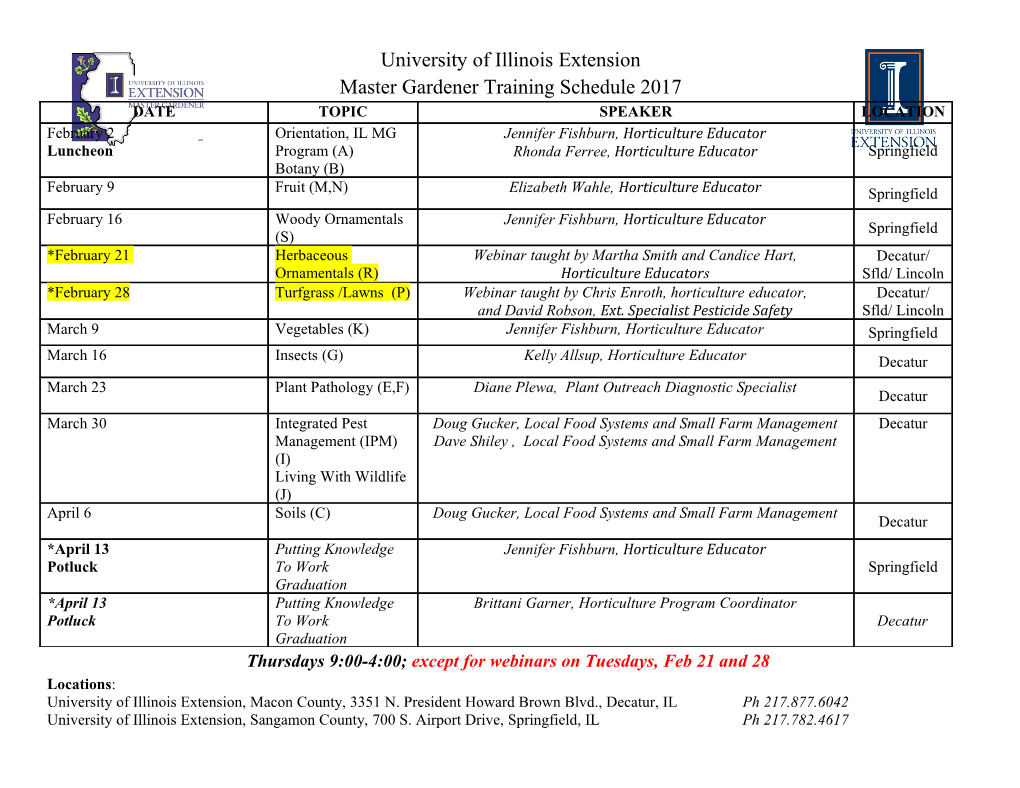
Carbon/Sulfur Nanocomposites and Additives for High-Energy Lithium Sulfur Batteries Chengdu Liang Oak Ridge National Laboratory Vehicle Technologies Program Annual Merit Review and Peer Evaluation Meeting, May 2012 Contributors: Zhan Lin, Nancy Dudney, and Jane Howe Project ID: ES105 “This presentation does not contain any proprietary, confidential, or otherwise restricted information” Managed by UT-Battelle for the Department of Energy Overview • Timeline • Budget – Start June, 2010 – $220k FY10 – $350k FY11 – $350k FY12 • Technical barriers for EV and PHEV – Low energy density • Partners – High cost of materials and – Oak Ridge National Laboratory processing – Center for Nanophase Materials – Fundamental research of high- Sciences, ORNL energy materials for Li-S – High Temperature Materials Lab, batteries ORNL • In situ SEM 2 Managed by UT-Battelle for the Department of Energy Objectives and relevance • Objectives: – Improve the electronic conductivity of sulfur by using high surface area mesoporous carbon materials – Extend the cycle-life Li-S batteries by creating C-S nanocomposites with protective layers – Explore electrolyte additive to catalyze the electrochemical reaction of Li2S • Relevance: – Enables high-energy Li-S battery chemistry for EV and PHEV batteries – Addresses the cost barriers for automobile batteries 3 Managed by UT-Battelle for the Department of Energy Milestones Milestones: Target: 1. Confirm the earlier observation of long cycle life in Sep, 2010 half cells and expand the synthesis of sulfur/carbon composite materials of various sulfur loading 2. Compare the performance for different Jan, 2011 concentrations of additives to the electrolyte 3. Investigate additives to the cathode, including Sep, 2011 catalysts and alternative sulfur compounds 4. Design new liquid electrolytes, considering both Sep, 2011 poor/good solvents for Li polysulfides 5. Synthesize novel composite cathodes to improve Sep, 2012 cyclability Sep, 2012 6. Explore full cell configuration to minimize excess lithium at the anode 4 Managed by UT-Battelle for the Department of Energy Approach to fundamental research of Li-S batteries. Goal: Develop advanced materials for Li-S batteries • Design novel composite materials for high energy sulfur cathode − C/S composite materials with optimized porous structures − Solid electrolyte coatings to retain sulfur at the cathode • Tailor electrolytes for Li-S batteries − Reduce the polysulfide shuttle − Electrolyte additives to catalyze the electrochemical reactions of Li2S • Explore approaches for anode protection − Suppress dendritic growth for Li-plating − Passivation of Li with designed SEI • Develop advanced diagnosis tools for the elucidation of battery reaction mechanisms − In situ SEM − Air-sensitive-sample handling 5 Managed by UT-Battelle for the Department of Energy Technical Progress – next 11 slides. Porosity is key to capacity retention. Double templating method has been developed to synthesize porous carbon with controlled porosity: • Two pore sizes were obtained by using PEO-PPO-PEO based triblock copolymers (7nm) and silica nanospheres (13nm) • Total porosity was controlled by the ratio of carbon precursors to the 1400 templates: two porosities of 1.12 and 3 100 2.35 cm /g. were demonstrated 1200 Pore volume 2.35 ) -1 1000 80 The C/S composites with 70 wt.% of sulfur loading showed that the sample 800 60 mAh g ( with large pore volume has better 600 40 capacity retention than the one with small 400 Charge, pore volume 2.35 pore volume. Capacity Discharge, pore volume 2.35 20 200 Charge, pore volume 1.12 Discharge, pore volume 1.12 (%) Coulombic Efficiency 0 0 5 10 15 20 6 Managed by UT-Battelle for the Department of Energy Cycle Number Polysulfide shuttle is still the bottle-neck of cycle-life. 3.2 0.5 3.0 ) -2 ) + 2.8 0.4 Before cycling mA cm 2.6 ( 0.3 2.4 V vsV Li/Li ( 2.2 Discharge 0.2 Charge 2.0 Voltage 0.1 1.8 Current Density After cycling 1.6 0.0 0 200 400 600 800 1000 1200 1400 Capacity (mAh g-1) The first charge and discharge curves SEM of the lithium anode before and of the C/S composite electrode with a after cycling: lithium sulfide deposition pore volume of 2.35 cm3/g. Two was observed on the lithium anode plateaus were observed with a ratio of • Polysulfide shuttle still exist in large 3:1 of the high to low plateaus. Current pore volume C/S composite tailing at the end of charging indicates • Capacity decay could be linked with possible polysulfide shuttle. sulfur loss through polysulfide shuttle. 7 Managed by UT-Battelle for the Department of Energy Solid electrolyte coated cathode for Li-S batteries. Li2S core A new core-shell structure overcomes the key challenges for Li-S cells: • Poor ionic and electronic conductivities • Low utilization of sulfur • Sulfur dissolution • Polysulfide shuttle • Low coulombic efficiency e • Corrosion of Li anode Li+ graphene • Poor cycling performance • Change of microstructure Concept: • Impart ionic and electronic conductivities to the cathode through intimate contacting with conductors Solid electrolyte shell • Prohibit sulfur dissolution and shuttle prevent direct contact of phenomenon by solid electrolyte coating Li2Sx with liquid electrolyte 8 Managed by UT-Battelle for the Department of Energy Li2S@Li4P2S7 Core-shell Nanoparticles were synthesized through facile solution chemistry approach. D=13 nm 111 220 Intensity (a. u.) 200 311 222 10 20 30 40 50 60 2θ (degrees) SEM of solid electrolyte coated XRD pattern of Li2S@Li4P2S7 electrode with a composition of 60 Core-shell Nanoparticles: particle wt.% Li4P2S7 coated Li2S size of Li2S was 13 nm based on nanoparticles, 30 wt.% carbon peak width. Li4P2S7 coating was black, 10 wt.% PVC confirmed by Raman spectrum. The coating was too thin to show significant XRD peaks 9 Managed by UT-Battelle for the Department of Energy Solid electrolyte coated cathode has superior cycling performance in liquid electrolytes. 3.2 40 Charge • The cathode ) 3.0 + Discharge consisted of pre- 2.8 30 ) lithiated nanoparticles A 2.6 µ ( • No shuttle V vs. Li/Li vs. V ( 2.4 20 phenomenon was 2.2 Current observed in any cell Voltage 2.0 10 • No sulfur dissolution 1.8 observed in the 1.6 0 0 200 400 600 800 1000 1200 electrolytes (good 1100 Capacity (mAh g-1) solvents, DME, 1000 charge discharge TEGDM) 900 • Capacity varies with 800 700 the electrode 600 preparation from 200 500 mAh/g to 1100 mAh/g 400 Capacity (mAh) 300 • Close to 100% 200 coulombic efficiency 100 0 0 5 10 15 20 Cycle number 10 Managed by UT-Battelle for the Department of Energy P2S5 as novel electrolyte additive for Li-S batteries. The photo presents the solubility of a series of mixtures of Li2Sx (1≤x≤8) with and without P2S5 in TEGDME P2S5 forms complex with Li2Sx and facilities the dissolution of lithium sulfide/polysulfides in ether based electrolytes. 200 Li2S/P2S5 Cyclic voltammograms (CV) of Li2Sx/P2S5 Li S /P S 150 2 2 2 5 complexes: Li2S4/P2S5 100 Li S /P S • No redox peak was observed in the Li2S/P2S5 2 6 2 5 A) Li S /P S µ 2 8 2 5 complex between 1.7 and 3.2 V. This complex I ( 50 can be reduced at potential below 1.7 V and be oxidized at potentials over 3.2 V. 0 • Complexes of Li2Sx/P2S5 (x≥2) have redo peaks -50 between 1.7 and 3.2 V. -100 1.5 2.0 2.5 3.0 3.5 4.0 11 Managed by UT-Battelle for the Department of Energy E (V) P2S5 improves cell performance. Capacity (mAh g-1) 1200 1000 800 600 400 200 0 3.2 1600 3.0 100 with P2S5 2.8 ) Discharge -1 1400 2.6 ) Charge without P2S5 + 80 2.4 1200 2.2 mAh g ( Li/Li 2.0 1000 with P S 1.8 2 5 60 vs. ( 1.6 800 1.4 1.2 600 40 1.0 Voltage 0.8 400 without P S 0.6 2 5 20 0.4 B 200 Coulombic efficiency (%) 0.2 Discharge capacity 0.0 0 A 0 0 200 400 600 800 1000 1200 0 5 10 15 20 25 30 35 40 -1 Capacity (mAh g ) Cycle number Charge discharge curves showed that Comparison of cycling performance of the polysuflide shuttle was suppressed: cells with/without P2S5. Much higher Nonporous carbon was used to capacity retention was observed in the demonstrate the efficacy of additive cell with the additive. effect. 12 Managed by UT-Battelle for the Department of Energy P2S5 passivates the surface of Li anode. SEM images of (A) the surface of pure metallic Li, (B, C) the passivation layer morphologies with different magnifications, and (D) the cross- section of the passivation layer peeled off from Li surface after the 1st cycle when using Li2S8/P2S5 as the catholyte in 1 m LiTFSI @ TEGDME. 13 Managed by UT-Battelle for the Department of Energy Composition of surface passivation layer on Li anode. Li (002) 418 XRD Raman Li (011) The surface pasivation layer is Li3PS4 Peaks matchs Intensity (a.u.) Intensity (a. u.) 184 Li3PS4 305 229 257 10 20 30 40 50 60 500 450 400 350 300 250 200 150 -1 2θ (Degrees) Wavelength (cm ) The function of the additive is two-fold: – P2S5 promotes the dissolution of Li2S and alleviates the loss of capacity caused by the precipitation of Li2S; and – P2S5 passivates the surface of lithium metal and therefore eliminates the polysulfide shuttle phenomenon. 14 Managed by UT-Battelle for the Department of Energy Air-transfer sample holder for cell diagnosis. A new sample transfer stage has been developed for taking samples from glovebox to a scanning electron microscope (SEM) without exposure to air and moisture.
Details
-
File Typepdf
-
Upload Time-
-
Content LanguagesEnglish
-
Upload UserAnonymous/Not logged-in
-
File Pages20 Page
-
File Size-