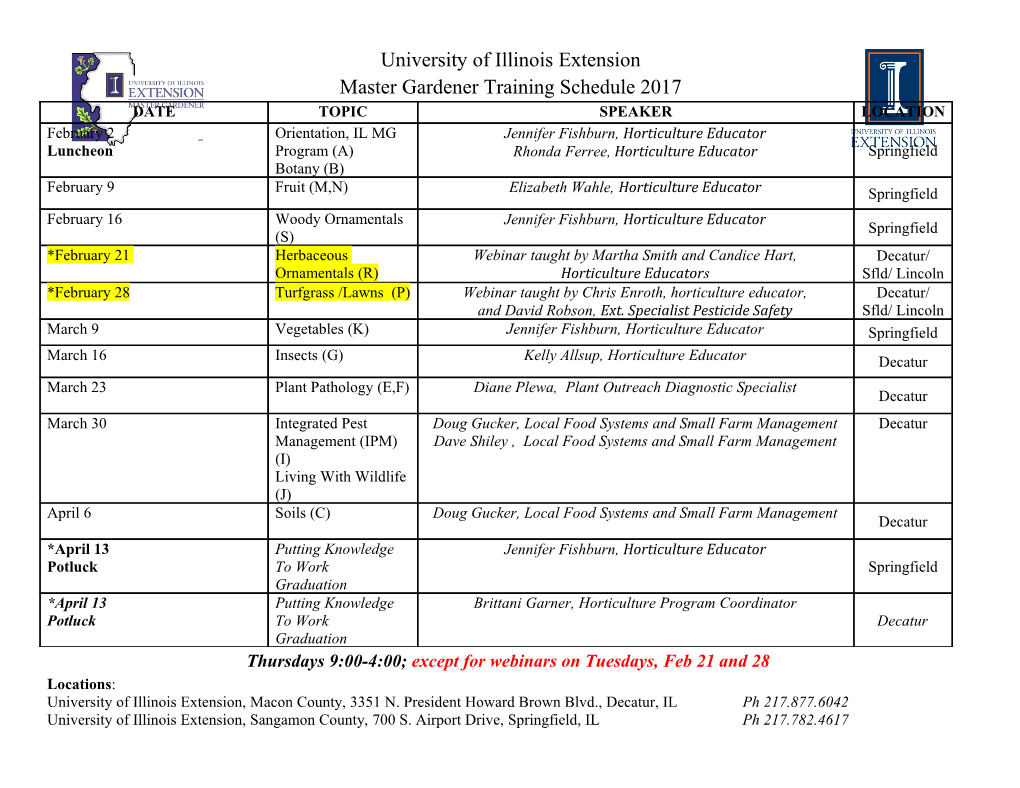
Dynamics of hydrocarbon cycling by microorganisms in the Gulf of Mexico Olivia Mason Florida State University Timeline of Findings (initial papers on microbial response) A Persistent Oxygen Anomaly Reveals the Fate of Spilled Methane in the Deep Gulf of Mexico (Science) Kessler, JD, et al. Deep-Sea Oil Plume Enriches Indigenous Oil- Key findings: Within ~ 120 days all of the Degrading Bacteria (Science) methane released,,p at plume de p,pth, was Hazen, TC, et al, and Mason, OU. respired by methanotrophic bacteria. Key findings: Gammaproteobacteria, particularly the Within this time the dominant bacteria reported order Oceanospirillales, dominate deep-sea plume. by Hazen, et al. and Valentine, et al. was Biodegradation ½ lives on the order of 1.2 to 6.1 supplanted by methanotrophs. days. May-June 2010 June 2010 April 20, 2010 May-June 2010 September-October 2010 Tracking Hydrocarbon Plume Propane Respiration Jump-Starts Transport and Biodegradation at Microbial Response to a Deep Oil Spill DHiDeepwater Horizon (SiScience ) (SiScience) Camilli, R, et al. Valentine, DL, et al Key findings: Tracked and reported Key findings: Propane and ethane, but not deep-sea plume. Dissolved oxygen methane, fuels a low diversity bloom of concentrations in deep-sea plume gammaproteobacteria (Cycloclasticus, suggest that hydrocarbons did not fuel Colwellia, and Oceanospirillaceae)inthe) in the appreciable microbial respiration. plume. Succession in microbial community structure Species richness in the deep-sea plume 1400 Uncontaminated sample pyrotag Proximal plume pyrotag Distal plume pyrotag 1200 1000 800 f OTUs f OTUs oo 600 Proximal plume station Number ~ 2 hr 400 sampled within a few hours of hydrocarbon input from 200 ~ 24 hr the plume . Species richness 0 decreased rapidly. 300 700 1100 1500 1900 2300 2700 3100 3500 3900 4300 4700 5100 5500 Number of Sequences Distal station sampled right 600 after the failed top kill effort . Uncontaminated sample metagenome Proximal plume metagenome Distal plume metagenome Species richness decreased 500 within in ~24 h. 400 cies ee These findings were 300 consistent with different ~ 2 hr methodologies. 200 ~ 24 hr mber of Observed Sp Observed of mber Nu 100 0 1000 6000 11000 16000 21000 26000 31000 36000 41000 46000 Number of Sequences Mason, et al (2012) Microbial community composition a 100 Proteobacteria;Oceanospirillales 90 Other RidhRapid change in mi crobi bilal 80 Proteobacteria;Colwellia community structure 70 Crenarchaeota;Nitrosopumilus towards the dominance of a 60 Proteobacteria;Cycloclasticus ance (%) ance (%) single, uncharacterized, dd Bacteria;Marine group A 50 uncultured Proteobacteria;Thalassomonas 40 Proteobacteria;Deltaproteobacteria Oceanospirillales OTU. 30 Proteobacteria;Gammaproteobacteria Relative abun 20 Euryy;gparchaeota;Marine group II Analysis of both Proteobacteria;Candidatus 10 metagenomic and Pelagibacter 0 metatranscriptomic Uncontaminated sample Proximal plume Distal plume b 100 sequence data agreed with 90 pyrotag data. First time the Gammaproteobacteria; 80 Oceanospirillales active community Other 70 characterized during the 60 Gammaproteobacteria; spill. ce (%) nn Alteromonadales 50 Deltaproteobacteria; NB1-j Oceanospirillales abundant 40 Gammaproteobacteria; Pseudomonadales and active, but what was it 30 Gammaproteobacteria; whole and other members of the elative abunda elative 20 genome shotgun isolate str. KT 71 RR Gammaproteobacteria; SAR86 microbial community 10 doing? 0 Uncontaminated Proximal Proximal Distal Distal 10 sample plume plume plume plume DNA cDNA DNA cDNA DNA Hydrocarbon degradation (whole community) Metagenomes Metatranscriptomes Toluene a b Toluene PAH PAH Ring cleavage/hydroxylating Ring cleavage/hydroxylating Benzene Benzene Aromatic Aromatic Ethylbenzene Ethylbenzene Particulate methane monooxygenase Particulate methane monooxygenase Cyclohexanone Cyclohexanone Benzoate Benzoate Cyyygclohexanol dehydrogenase Cyyygclohexanol dehydrogenase n-alkane n-alkane Fatty acid Fatty acid Aldehyde dehydrogenase Aldehyde dehydrogenase Alcohol dehydrogenase Alcohol dehydrogenase 0.00001 0.0001 0.001 0.01 0.1 000010.0001 00010.001 0010.01 010.1 Uncontaminated station Proximal plumestation Distal plume station Two abundant pathways identified in both potential and expressed datasets: • n-alkane oxidation (alkane monooxygenase -> alcohol and aldehyde dehydrogenases -> beta oxidation) • cyclohexane oxidation (alkane monooxygenase -> cyclohexanone monooxygenase -> cyclohexanol dehydrogenase -> beta oxidation) • Note the low abundance of genes and transcripts involved in PAH and BTEX degradation. Metabolic reconstruction of the dominant Oceanospirillales Siderophore Type II secretion production system Exopolysaccharide Cyclohexane Silver transport Arsenite* Cobalt/ Cadmium/Zinc MCP Peptidases Alkane monooxygenase* ß-oxidation - Cyclohexanol Tonß Flagellum HO Cyclohexanol dehydrogenase Chemotaxis Cyclohexanone monooxygenase = Cyclohexanone Ammonia O Carboxylic acids Iron Fatty acids Sulfate Amino acids Phosphate Manganese/ Cobalt/ Sodium/ Zinc Cadmium/Zinc proline import Fi 5 Uncultured Oceanospirillales single cell draft genome (1. 9 Mb) has genes coding for: 1) Motility 2) Cyclohexane degradation 4) Nutrient acquisition These genes were abundant in the plume metagenomes and metatranscriptome. Oceanospirillales continues to elude cultivation efforts. Likely due to its metabolism of cyclohexane. June 2010: Propane Respiration Jump-Starts Microbial Response to a Deep Oil Spill Propane, ethane, and possibly butane fuel microbial response, but not methane, despite its abundance. Colwellia the dominant microbe in the plume. Shown to incorporate ethane, propane, and benzene. Some methane, bhlbut much less. Valentine et al. Science 2010;330:208-211 Sequenced genome of Colwellia ppysychrer ythraea provided few clues about its role in hydrocarbon degradation, so what were the dominant Colwellia doing in the plume? Redmond and Valentine(2012) Single cell genome sequence of Colwellia Dominant Colwellia has a butane monooxygenase! It is capable of methane oxidation, but preferentially degrades butane , ethane and propane. This finding explains why methane didn ’t fuel respiration in Butane the plume when Colwellia H 2 CH C 3 abundant. C H3C H2 Butane monooxygenase ß-oxidation MCP OH H 2 CH C 2 H O 1-Butanol 2 H C C C 3 H Butyric Acid OH 2 Flagellum H C C 3 H 2 Alcohol dehydrogenase Aldehyde dehydrogenase O H2 C CH Butyraldehyde C H3C H2 Sept-Oct 2010: A Persistent Oxygen Anomaly Reveals the Fate of Spilled Methane in the Deep Gulf of Mexico • No CH4 but methylotrophic bacteria were detected at each site (5 and 36%). • In Sept 2010 both methane concentrations and methane oxidation rates were very low, suggesting that methanotrophs were no longer active** and that we only observed the remnants of that population . • We suggest that a vigorous deepwater bacterial bloom respired nearly all the released methane within this time [120 d]dhbdays], and that by ana logy, large-scale releases of methane from hydrate in the deep ocean are likely to be met by a Stations are shown from left to right in order of similarlyyp rapid methanotrophic decreasing reductions in DO. Collected in response. September. n = 56 to 79 per station for a total of 492 samples. ((gcoverage is veryy) low) J D Kessler et al. Science 2011;331:312-315 Published by AAAS Hydrocarbon cycling by microorganisms in the Gulf of Mexico water column • All methane in 120 days .( !) Chla (µg/L) 0 0.2 0.4 0.6 0 0.2 0.4 0.6 0 0.2 0.4 0.6 • However, known methane oxidizers not CH4 (nM) and O2 (mg/L) 3 5 7 9 11 13 15 3 5 7 9 11 13 15 3 5 7 9 11 13 15 abundant until after the spill ended. 0 100 200 300 400 WB1306 WB1305 WB1305 • Mason et al (2012) used shotgun 500 CT15 CT32 CT21 600 Peanut Seep Seep C Seep A metatranscriptomics and showed that while 700 800 known methanotrophs were not abundant (less 900 1000 than 1%) early in the spill, active methane 1100 1200 oxidation was occurring . 1300 1400 1500 1600 1700 • Goal: to better understand hydrocarbon, and 1800 1900 particularly, methane cycling by 2000 0 0.2 0.4 0.6 0 0.2 0.4 0.6 0 0.2 0.4 0.6 microorganisms in the Gulf using “iTag” 3 5 7 9 11 13 15 3 5 7 9 11 13 15 3 5 7 9 11 13 15 0 sequencing (who is there?) and ‘omics’ 100 200 techniques (what are doing?). 300 400 WB1305 WB1306 WB1306 500 600 CT12 CT17 CT23 700 AC2 Seep C2 AC5 800 900 1000 1100 1200 1300 1400 1500 1600 1700 Chla 1800 Oxygen 1900 2000 Methane Deep-C cruises September and October 2012 Water column: distribution of methane-oxidizing Bacteria Depth (m) CH 4 CH Chla 4 NMDS2 PO NMDS2 4 NO3 Methanotrophs (Methylococcales) NMDS2 • Very low abundance of known methanotrophs throughout water column: – thus, methane biofilter remains enigmatic • Greater diversity of methanotrophs than is currently recognized? Methane production in the oceanic photic zone: microbial community structure Methane Chlorophyll a Oxygen Phosphate Nitrate - Chla and CH4 (r = 0.80) -O2 and CH4 (r = 0.28) -PO4 and NO3 with CH4 (r = -070and0.70 and -0680.68, respectively) • Methane production is an anaerobic process, yet methane is produced in the aerobic surfidlThiilldth‘face mixed layer. This is called the ‘oceanithdic methane paradox.’ • Current hypothesis: methylphosphonate (MPn) cycling leads to methane production when P is limiting: • MPn produced by Nitrosopumilus maritimus, Cyanobacteria? • Many marine microbes can use MPn, including Cyanobacteria. Community structure at chlorophyll/methane
Details
-
File Typepdf
-
Upload Time-
-
Content LanguagesEnglish
-
Upload UserAnonymous/Not logged-in
-
File Pages15 Page
-
File Size-