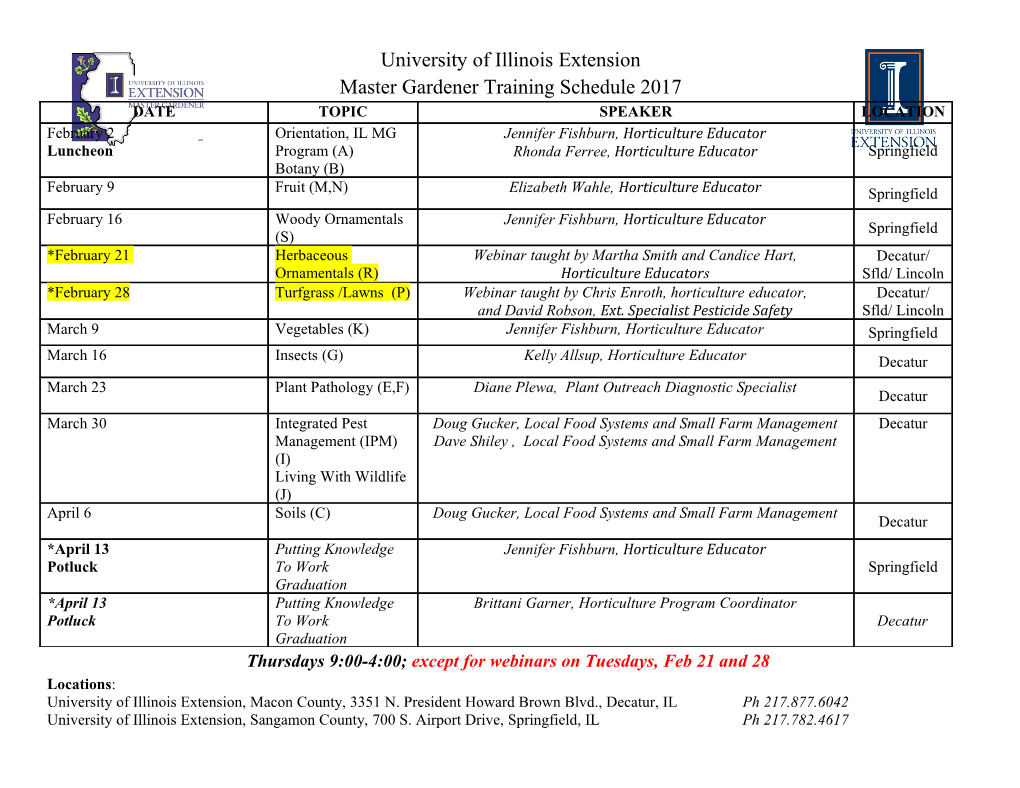
Supporting Information Wilson et al. 10.1073/pnas.0804276105 Fig. S1. Sites of oxazolidinone resistance mutations in bacteria and archaea. (a) Secondary structure of the peptidyltransferase ring of the 23S rRNA from D. radiodurans with the mutation sites in various bacteria (1–5) indicated with blue lettering. Shaded numbers indicate nucleotides that directly interact with linezolid in the D50S structure. (b) Secondary structure of the peptidyltransferase ring of the 28S rRNA from H. sapiens with the mutation sites in various bacteria and archaea (from a and c) indicated with blue lettering. Shaded numbers indicate nucleotides that directly interact with linezolid in the D50S structure. Red lettering indicates positions where the H. sapiens 28S rRNA nucleotides have the equivalent base to oxazolidinone resistant bacteria or archaea. (c) Secondary structure of the peptidyltransferase ring of the 23S rRNA from H. marismortui with the mutation sites in various archaea (6) indicated with blue lettering. Shaded numbers indicate nucleotides that directly interact with linezolid in the D50S structure. (d) Secondary structure of the peptidyltransferase ring showing the conservation of this region across all three kingdoms (excluding organelles). Capital or lowercase red letters indicate 98%ϩ% and 90–98% conservation whereas closed and open circles represent 80–90% and Ͻ80%, respectively. Shaded numbers indicate nucleotides that directly interact with linezolid in the D50S structure. Diagrams adapted from the comparative RNA web site (www.rna.ccbb.utexas.edu) (7). 1. Sander P, et al. (2002) Ribosomal and non-ribosomal resistance to oxazolidinones: species-specific idiosyncrasy of ribosomal alterations. Mol Microbiol 46:1295–1304. 2. Xiong L, et al. (2000) Oxazolidinone resistance mutations in 23S rRNA of. Escherichia coli reveal the central region of domain V as the primary site of drug action J Bacteriol 182:5325–5331. 3. Tsiodras S, et al. (2001) Linezolid resistance in a clinical isolate of. Staphylococcus aureus Lancet 358:207–208. 4. Prystowsky J, et al. (2001) Resistance to linezolid: characterization of mutations in rRNA and comparison of their occurrences in vancomycin-resistant enterococci. Antimicrob Agents Chemother 45:2154–2156. 5. Swaney SM, Aoki H, Ganoza MC, Shinabarger DL (1998) The oxazolidinone linezolid inhibits initiation of protein synthesis in bacteria. Antimicrob Agents Chemother 42:3251–3255. 6. Kloss P, Xiong L, Shinabarger DL, Mankin AS (1999) Resistance mutations in 23 S rRNA identify the site of action of the protein synthesis inhibitor linezolid in the ribosomal peptidyl transferase center. J Mol Biol 294:93–101. 7. Cannone JJ, et al. (2002) The comparative RNA web (CRW) site: an online database of comparative sequence and structure information for ribosomal, intron, and other RNAs. BioMed Central Bioinformatics 3:2. Wilson et al. www.pnas.org/cgi/content/short/0804276105 1of6 Fig. S2. Comparison of electron density between native and linezolid D50S structures. (a) 2Fo-Fc density map contoured at 1.5 sigma for the native D50S indicating the lack of density for the base of U2585 as well as within the linezolid binding pocket. The position of linezolid (red) in the D50S-linezolid structure is shown for reference. A putative magnesium ion in the native structure that overlaps the binding position of linezolid is indicated by a blue sphere. (b–d)2Fo Ϫ Fc density map contoured at 1.5 (b),2(c) and 2.5 (d) sigma for the D50S-linezolid structure, revealing the additional density for the drug and the base of U2585. The modeled position of linezolid (red) and U2585 in the D50S-linezolid structure is as indicated. A putative magnesium ion that can be coordinated by the fluorine of ring B and the tail of linezolid is shown as a blue sphere. Wilson et al. www.pnas.org/cgi/content/short/0804276105 2of6 Fig. S3. Comparison of different oxazolidinones modeled into the linezolid binding pocket. (a) Reference position of linezolid (red) within the binding pocket formed by eight universally conserved nucleotides (blue). (b and c) Regularized models for the binding of oxazolidinones used in the crosslinking study of Leach et al. (1). (b) The photoreactive sidechain of XL1 can potential stack on the base of G2505 consistent with crosslinking observed to this position. (c) The photoreactive sidechain of XL3 can stack on the base of A2602 within the E. coli 70S structure (PDB ID code 2AW4; blue) (2), however, in the native and linezolid-D50S structure, this base is located in a different position (red). (d–f) Comparison of the position of U2585 in the D50S-linezolid structure (red) (f), with U2585 from regularized models of ranbezolid (teal) (d) and a tricyclic analog (orange) (e). Potential hydrogen bonding (dashed lines) and stacking interactions (arrowed) are indicated. (g) Comparison in the positions of U2585 in the linezolid-D50S (red) with the quinupristin (streptogramin B) bound D50S structure (PDB ID code 1SM1; gray) (3). (h) Comparison in the positions of U2585 in the linezolid-D50S (red) and H50S bound with A- (yellow) and P-site (orange) CCA-end mimics (PDB ID code 1VQN; blue) (4). 1. Leach KL, et al. (2007) The site of action of oxazolidinone antibiotics in living bacteria and in human mitochondria. Mol Cell 26:393–402. 2. Schuwirth B, et al. (2005) Structures of the bacterial ribosome at 3.5 Å resolution. Science 310:827–834. 3. Harms J, et al. (2004) Alterations at the peptidyl transferase centre of the ribosome induced by the synergistic action of the streptogramins dalfopristin and quinupristin. BMC Biol 2:4. 4. Schmeing TM, Huang KS, Strobel SA, Steitz TA (2005) An induced-fit mechanism to promote peptide bond formation and exclude hydrolysis of peptidyl-tRNA. Nature 438:520–524. Wilson et al. www.pnas.org/cgi/content/short/0804276105 3of6 Fig. S4. Influence of U2504 on linezolid and anisomycin binding. (a) Difference in the position of U2504 (arrowed) and linezolid between the D50S-linezolid (pink) and H50S-linezolid (orange) structures (U.S. patent number 6,947,845 B2). (b) The binding position of anisomycin on the H50S (PDB ID code 1K73; green) (1) is incompatible with the orientation of U2504 in the D50S (pink) structures. 1. Hansen JL, Moore PB, Steitz TA (2003) Structures of five antibiotics bound at the peptidyl transferase center of the large ribosomal subunit. J Mol Biol 330:1061–1075. Wilson et al. www.pnas.org/cgi/content/short/0804276105 4of6 Fig. S5. Oxazolidinones induce an A/O state recognized by LepA. (a) Relative position of linezolid (red), P-tRNA (cyan), A-tRNA (pale green), LepA (maroon density and ribbon) and A/L-tRNA (blue). Boxed region (right) shows an enlargement illustrating the overlap between linezolid (red) and the positionofan A-tRNA (pale green), but compatibility with the A/L-tRNA (blue) position. A- and P-tRNA are constructed from (1, 2) and LepA and A/L-tRNA from (3, 4). (b) Model illustrating that oxazolidinones (red) prevent accommodation of A-tRNA (green) at the A site of PTC of the 50S subunit, inducing an A/O state. We hypothesize that the A/O state is recognized LepA, which binds to the ribosome and stabilizes the A/L state. 1. Schmeing TM, Huang KS, Strobel SA, Steitz TA (2005) An induced-fit mechanism to promote peptide bond formation and exclude hydrolysis of peptidyl-tRNA. Nature 438:520–524. 2. Yusupov MM, et al. (2001) Crystal structure of the ribosome at 5.5 A resolution. Science 292:883–896. 3. Connell SR, et al. (2008) A novel tRNA-intermediate revealed on the ribosome during EF4-mediated back-translocation. Nat Struct Mol Biol, in press. 4. Evans RN, Blaha G, Bailey S, Steitz TA (2008) The structure of LepA, the ribosomal back translocase. Proc Natl Acad Sci USA 105: 4673–4678. Wilson et al. www.pnas.org/cgi/content/short/0804276105 5of6 Table S1. Table of crystallographic and refinement data Crystal Information Space group I222 Unit cell dimensions, Å 169.7 ϫ 410.6 ϫ 695.0 Resolution, Å 30–3.50 (3.77–3.50) No. of unique reflections 272,947 (45,746) Completeness, % 90.5 (80.2) Rsym, % 13.3 (45.7) Mean I/s(I) 8.2 (1.9) Refinement statistics R factor, % 25.9 (30.9) Rfree, % 28.0 (32.0) Wilson B-factor 34.3 RMS deviations from ideal geometry Bond length 0.010 Bond angles 1.5 Dihedral angles 19.6 Improper angles 1.73 Wilson et al. www.pnas.org/cgi/content/short/0804276105 6of6.
Details
-
File Typepdf
-
Upload Time-
-
Content LanguagesEnglish
-
Upload UserAnonymous/Not logged-in
-
File Pages6 Page
-
File Size-