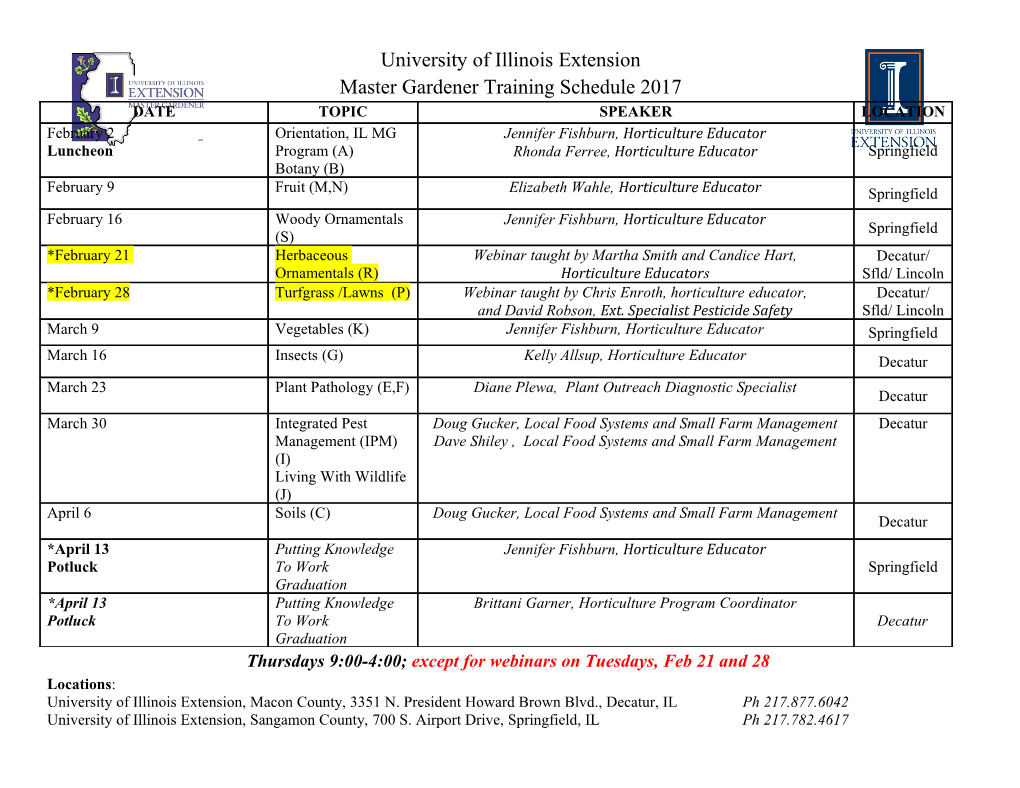
bioRxiv preprint doi: https://doi.org/10.1101/858514; this version posted April 15, 2020. The copyright holder for this preprint (which was not certified by peer review) is the author/funder. All rights reserved. No reuse allowed without permission. 1 Charting the metabolic landscape of the facultative methylotroph Bacillus 2 methanolicus 3 4 Baudoin Delépinea, Marina Gil Lópezb, Marc Carnicera*, Cláudia M. Vicentea, Volker F. 5 Wendischb, Stéphanie Heuxa# 6 7 a TBI, Université de Toulouse, CNRS, INRA, INSA, Toulouse, France. 8 b Genetics of Prokaryotes, Faculty of Biology & CeBiTec, Bielefeld University, Bielefeld, 9 Germany 10 11 Running Head: Metabolic states of the methylotroph B. methanolicus 12 13 # Address correspondence to SH, [email protected] 14 Post: TBI - INSA de Toulouse, 135 avenue de Rangueil, 31077 Toulouse CEDEX 04; Phone 15 number: +33 (0)5 61 55 93 55 16 * Present address: IQS, Universitat Ramon Llull, Via Augusta 390, E-08017, Barcelona, Spain 17 1 bioRxiv preprint doi: https://doi.org/10.1101/858514; this version posted April 15, 2020. The copyright holder for this preprint (which was not certified by peer review) is the author/funder. All rights reserved. No reuse allowed without permission. 18 ACKNOWLEDGEMENT 19 MetaToul (www.metatoul.fr), which is part of MetaboHub (ANR-11-INBS-0010, 20 www.metabohub.fr) are gratefully acknowledged for their help in collecting, processing and 21 interpreting 13-C NMR and MS data. We would like to thank Marcus Persicke, Maud Heuillet, 22 Edern Cahoreau, Lindsay Periga, Pierre Millard, Gilles Vieira and Jean-Charles Portais for the 23 insights they provided. 24 25 FUNDING 26 This work was supported by ERA-CoBioTech’s project C1Pro (ANR-17-COBI-0003-05 and 27 FNR-22023617). 28 29 30 31 32 33 34 35 36 2 bioRxiv preprint doi: https://doi.org/10.1101/858514; this version posted April 15, 2020. The copyright holder for this preprint (which was not certified by peer review) is the author/funder. All rights reserved. No reuse allowed without permission. 37 ABSTRACT 38 Bacillus methanolicus MGA3 is a thermotolerant and relatively fast-growing methylotroph able 39 to secrete large quantities of glutamate and lysine. These natural characteristics make B. 40 methanolicus a good candidate to become a new industrial chassis organism, especially in a 41 methanol-based economy. This has motivated a number of omics studies of B. methanolicus at 42 the genome, transcript, protein and metabolic levels. Intriguingly, the only substrates known to 43 support B. methanolicus growth as sole source of carbon and energy are methanol, mannitol, and 44 to a lesser extent glucose and arabitol. We hypothesized that comparing methylotrophic and non- 45 methylotrophic metabolic states at the flux level would yield new insights into MGA3 46 metabolism. 13C metabolic flux analysis (13C-MFA) is a powerful computational method to 47 estimate carbon flows from substrate to biomass (i.e. the in vivo reaction rates of the central 48 metabolic pathways) from experimental labeling data. In this study, we designed and performed 49 a 13C-MFA of the facultative methylotroph B. methanolicus MGA3 growing on methanol, 50 mannitol and arabitol to compare the associated metabolic states. The results obtained validate 51 previous findings on the methylotrophy of B. methanolicus, allowed us to characterize the 52 assimilation pathway of one of the studied carbon sources, and provide a better overall 53 understanding of this strain. 54 IMPORTANCE 55 Methanol is cheap, easy to transport and can be produced both from renewable and fossil 56 resources without mobilizing arable lands. As such, it is regarded as a potential carbon source to 57 transition toward a greener industrial chemistry. Metabolic engineering of bacteria and yeast able 58 to efficiently consume methanol is expected to provide cell factories that will transform methanol 59 into higher-value chemicals in the so-called methanol economy. Toward that goal, the study of 3 bioRxiv preprint doi: https://doi.org/10.1101/858514; this version posted April 15, 2020. The copyright holder for this preprint (which was not certified by peer review) is the author/funder. All rights reserved. No reuse allowed without permission. 60 natural methylotrophs such as B. methanolicus is critical to understand the origin of their 61 efficient methylotrophy. This knowledge will then be leveraged to transform such natural strains 62 into new cell factories, or to design methylotrophic capability in other strains already used by the 63 industry. 64 KEYWORDS 65 Natural methylotrophy, 13C metabolic flux analysis, non-stationary MFA, Bacillus methanolicus 66 MGA3, methanol 67 4 bioRxiv preprint doi: https://doi.org/10.1101/858514; this version posted April 15, 2020. The copyright holder for this preprint (which was not certified by peer review) is the author/funder. All rights reserved. No reuse allowed without permission. 68 ABBREVIATIONS 69 13C-MFA: 13C metabolic flux analysis; CID: carbon isotopologue distribution; FBA: Flux 70 Balance Analysis; MS: mass spectrometry; NMR: nuclear magnetic resonance; PTS: 71 phosphotransferase system 72 Pathways 73 SBPase: fructose bisphosphate aldolase/sedoheptulose bisphosphatase; TA: fructose 74 bisphosphate aldolase/transaldolase; PPP: pentose phosphate pathway; RuMP: Ribulose 75 Monophosphate Cycle; TCA: Krebs cycle 76 Reactions 77 akgdh: 2-oxoglutarate dehydrogenase and 2-oxoglutarate synthase; Araupt: arabitol uptake; 78 detox: linear detoxification pathways; fum: fumarate reductase; glpx: sedoheptulose- 79 bisphosphatase; gnd: phosphogluconate dehydrogenase; hps: 3-hexulose-6-phosphate synthase ; 80 idh: isocitrate dehydrogenase; Manupt: mannitol uptake; mdh: methanol dehydrogenase; pdh: 81 pyruvate dehydrogenase; pfk: 6-phosphofructokinase and fructose-bisphosphatase; pgi: glucose 82 6-phosphate isomerase ; pgk: glyceraldehyde 3-phosphate dehydrogenase, phosphoglycerate 83 kinase, and phosphoglycerate mutase; phi: 6-phospho-3-hexuloisomerase ; pyk: pyruvate kinase; 84 rpe: ribulose-phosphate 3-epimerase; rpi: ribose 5-phosphate isomerase; rpi: ribulose phosphate 85 isomerase; ta: transaldolase; tkt1, tkt2: transketolases; zwf: glucose-6-phosphate dehydrogenase 86 and 6-phosphogluconolactonase. 87 Metabolites 88 Ace: acetate; Aco: aconitate; AKG: 2-oxoglutarate; ala: alanine; Ara: arabitol; arg: arginine; asp: 89 aspartate; Cit: citrate; DHAP: dihydroxyacetone phosphate; Ery4P: erythrose 4-phosphate; For: 5 bioRxiv preprint doi: https://doi.org/10.1101/858514; this version posted April 15, 2020. The copyright holder for this preprint (which was not certified by peer review) is the author/funder. All rights reserved. No reuse allowed without permission. 90 formaldehyde; Fru6P: fructose 6-phosphate; FruBP: fructose 1,6-bisphosphate; Fum: fumarate; 91 G3P: 3-phospho-D-glycerate; GAP: glyceraldehyde 3-phosphate; Glc6P: glucose 6-phosphate; 92 glu: glutamate; gly: glycine; GlyOx: glyoxylate; Gnt6P: 6-phosphogluconate; Hex6P: hexulose 93 6-phosphate; his: histidine; ile: isoleucine; leu: leucine; lys: lysine; Mal: malate; Man: mannitol; 94 MeOH: methanol; met: methionine; OAA: oxaloacetate; PEP: phosphoenolpyruvate; PGA: 2- 95 phospho-D-glycerate; phe: phenylalanine; pro: proline; Pyr: pyruvate; Rib5P: ribose 5- 96 phosphate; Ribu5P: ribulose 5-phosphate; Sed7P: sedoheptulose 7-phosphate; ser: serine; Suc: 97 succinate; thr: threonine; tyr: tyrosine; val: valine; Xyl5P: xylulose 5-phosphate. 98 6 bioRxiv preprint doi: https://doi.org/10.1101/858514; this version posted April 15, 2020. The copyright holder for this preprint (which was not certified by peer review) is the author/funder. All rights reserved. No reuse allowed without permission. 99 1. INTRODUCTION 100 13C metabolic flux analysis (13C-MFA) has emerged in the last decade as an outstanding 101 experimental method to describe the metabolic states of microorganisms. It has successfully been 102 used to identify new pathways (1), investigate responses to environmental changes (2), improve 103 the titer of cell factories (3), screen strains based on their enzymatic capacity (4), and more 104 generally to provide a better understanding of the metabolism of microorganisms (5) such as 105 methylotrophs (6-8). Briefly, 13C-MFA exploits a metabolic model and 13C-isotope patterns 106 measured from key metabolites to estimate reaction rates consistent with the observed labeling 107 patterns (see (9, 10) for reviews). Specifically, cells are grown on a 13C labeled carbon source, 108 metabolites of the central metabolism or constituents of the biomass such as proteinogenic amino 109 acids are sampled and quenched, and the labeling patterns of metabolites are monitored by mass 110 spectrometry (MS) and/or nuclear magnetic resonance (NMR). With NMR carbon isotopomers 111 are determined and hence positional information is provided whereas with MS, isotopologues are 112 identified. A metabolic model is then used to fit these measurements to theoretical data that are 113 simulated by optimizing reaction flux values from the metabolic model. Assuming mass balance, 114 if the measurements are coherent and the topology of the metabolic model is correct, the 115 experimental and simulated data will converge, yielding the estimated reaction fluxes. Finally, 116 13C-MFA provides a flux map: a predicted snapshot of the metabolic fluxes through the organism 117 of interest during the experiment, i.e. its metabolic state. 13C-MFA flux maps are conceptually 118 similar to those from flux balance analysis
Details
-
File Typepdf
-
Upload Time-
-
Content LanguagesEnglish
-
Upload UserAnonymous/Not logged-in
-
File Pages48 Page
-
File Size-