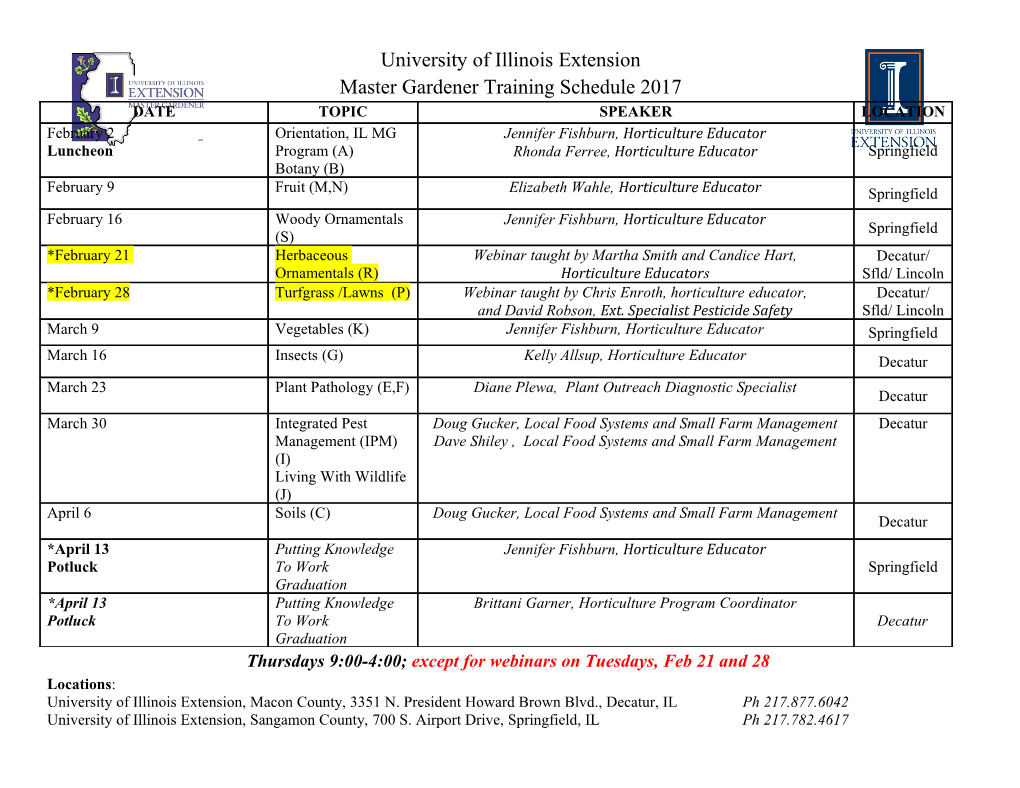
J Am Oil Chem Soc DOI 10.1007/s11746-015-2623-6 ORIGINAL PAPER Phase Behavior and Structure of Systems Based on Mixtures of n-Hentriacontane and Melissic Acid Lourdes Liliana Serrato-Palacios1 · Jorge F. Toro-Vazquez1 · Elena Dibildox-Alvarado1 · Antonio Aragón‑Piña2 · Maria del Rosario Morales-Armenta1 · Vrani Ibarra-Junquera3 · Jaime David Pérez‑Martínez1 Received: 14 October 2014 / Revised: 13 January 2015 / Accepted: 21 February 2015 © AOCS 2015 Abstract Candelilla wax (CW) organogelation is a prom- Introduction ising method for providing structure to solid-like fat-based foods, cosmetics, and drug delivery systems. The main com- Application of vegetable waxes to gel vegetable oil is ponent, n-hentriacontane (C31), defines, to a large extent, currently being explored to reduce the intake of fats rich the thermal properties of CW. CW is a complex mixture of in saturated and trans fatty acids [1]. Among the studied n-alkanes, fatty acids, triterpenic alcohols, and fatty alco- waxes, candelilla wax (CW) at concentrations higher than hols. In this initial examination of the role of CW compo- 1 % (w/w) develops vegetable oil organogels with recog- nents on the phase behavior and structure, CW and binary nized oil retention and textural properties that can produce mixtures of C31 and melissic acid (C30) were studied in solid-like water in oil emulsions [2, 3]. Some functional bulk and in solutions of vegetable oil. Binary mixtures of properties can be modulated by the addition of saturated C31 and C30 presented a predominantly monotectic phase TAGs (i.e., tripalmitin), as well as the application of shear- behavior in which the melting temperature of C31 remained ing during the gelation process [4]. According to previous constant independently of the mixture composition. Crys- reports, the main components of CW are n-alkanes, with tal packing of the pure components and mixtures had an n-hentriacontane (C31; 35.5 %) as the major constituent, orthorhombic subcell, and the microstructures of the mix- triterpenic alcohols (24.8 %), fatty acids with an even num- tures were extremely different from that of the pure compo- ber of carbons (16.6 %, of which 7.8 % is melissic acid), nents. In solutions with less than 50 % C31, the order–disor- fatty alcohols (3.61 %), and C39 to C64 esters (5.83 %) der transition observed in bulk C31 was absent. Organogels [3]. Despite the large number of components, CW phase of C31, C30, or the mixture were softer than those of CW. behavior is attributed almost exclusively to the C31 phase The increased strength of CW organogels is associated with transitions because both CW and C31 have an orthorhom- the relatively small crystal size of this system. bic subcell packing at room temperature [4, 5]. Within this context, it has been hypothesized that some CW compo- Keywords n-Hentriacontane · Melissic acid · Candelilla nents are incorporated into the C31 crystal lattice and that wax · Organogel others melt at temperatures close to the C31 melting point [3, 4]. Nonetheless, the type of solid phase structure (i.e., * Jaime David Pérez-Martínez solid solution, compound, solid dispersion) of CW remains [email protected] unknown. This information is fundamental to understand and tailor the functionality (e.g., melting profile, organo- 1 Facultad de Ciencias Químicas, Centro de Investigación gelling capacity, and rheological properties) of this natural y Estudios de Posgrado, Universidad Autónoma de San Luis Potosí, Av. Dr. Manuel Nava 6, Zona Universitaria, wax. 78210 San Luis Potosí, SLP, Mexico The phase behavior of long-chain n-alkanes and 2 Instituto de Metalurgia, Universidad Autónoma de San Luis fatty acids has been extensively studied, but mixtures Potosí, 78210 San Luis Potosí, Mexico of these compounds are largely unexplored. To the best 3 Bioengineering Laboratory, Universidad de Colima, of our knowledge only Benziane et al. [6] have studied 28400 Coquimatlán, Colima, Mexico this type of mixture and observed a eutectic behavior of 1 3 J Am Oil Chem Soc binary mixtures of n-eicosane (C20; m.p. 35.80 °C)/ system with the highest melting temperature; after 20 min = lauric acid (C12; m.p. 43.00 °C), n-octacosane (C28; at this temperature the sample was cooled (5 °C/min) until = m.p. 60.10 °C)/palmitic acid (C16; m.p. 60.60 °C), achieving 30 °C for 1 min, then the system was heated = = and n-tetracosane (C24; m.p. 51.3 °C)/stearic acid (C18; (5 °C/min) to 15 °C above the system melting temperature. = m.p. 69.65 °C) with eutectic mixtures (i.e., mixture with Using the first derivative of the heat flow, we calculated the = the lowest melting point) of x 0.55, x 0.4, and melting enthalpy, temperature at the onset and maximum C20 = C28 = x 0.85, respectively. That is, the solid phases of these of the endotherms with the equipment software (Universal C24 = n-alkane/fatty acid mixtures were dispersions of one crys- Analysis 2000 Ver. 4.2E; TA Instruments-Waters LLC). For talline entity rich in n-alkanes and one rich in fatty acids. each system, at least two independent determinations were It was also evident that all components displayed melting performed. temperature depression, but a differential behavior was observed as a function of the melting temperatures of pure Polarized Light Microscopy components in the binary mixture. A relevant aspect that has also been overlooked is the mixing impact of the sys- Microphotographs were obtained using a polarized light tem compositions on crystal microstructure. It is known microscope (BX5O; Olympus Optical Co, Ltd., Tokyo, that mixtures of stearic alcohol and stearic acid produce Japan) equipped with a digital color video camera (KPD50; smaller crystals than pure compounds [7, 8]. Thus, modu- Hitachi Digital, Tokyo, Japan), temperature-controlled lation of the crystal habit could be used to tailor rheologi- stage LTS 330, and temperature control station LTS350 cal properties and/or oil binding capacity of wax-based Linkam (Scientific Instruments, Waterfield, UK). Samples organogels. The aim of this investigation was to develop a of the systems (3 mg) were placed over a glass slide and phase diagram and study the microstructure of the binary heated for 20 min at the melting temperature determined system n-hentriacontane (C31)/triacontanoic acid (C30; using DSC, and a coverslip was placed on the molten sam- melissic acid) in bulk and in high oleic safflower oil solu- ple. Microphotographs of the systems were obtained after tions to model the candelilla wax crystal phase. 24 h of storage at 25 °C using the Linksys software version 1.3.1. (Linkam Scientific Instruments, Waterfield, UK). Materials and Methods Wide-angle X-ray Diffraction Refined CW was obtained from Multiceras (Monterrey, Wide-angle X-ray (WAX) diffraction patterns of pure com- Mexico), and C31 ( 99 % purity; Sigma) and C30 ( 98 % ponents and solutions were performed in an X-ray dif- ≥ ≥ purity; Fluka) were used as delivered. Mixtures were pre- fractometer (Panalytical, X’Pert Pro; Almelo, the Nether- pared in molar fraction ratios. Compounds were weighed lands) with CuKaR X-rays (λ 1.54 A) operating at 35 kV = and mixed in the sample container, heated to 10 °C above and 30 mA and a 0.5° divergence slit, 1° scatter slit, and the highest melting compound for 20 min, and mechani- 5.5 mm receiving slit. Bulk materials and organogels were cally stirred to obtain a compositionally homogeneous sys- developed and stored using the same thermal conditions as tem. To evaluate the solvent effect, the CW, pure compo- for microscopy. Prior to analysis, bulk samples were finely nents, and selected mixtures were added (4–90 % wt/wt) grated, and organogels were gently stirred to avoid pref- to high oleic safflower oil (HOSFO), heated to complete erential orientation of the crystallites. Diffraction patterns melting under constant stirring for 15 min, cooled to room were obtained by scanning the samples from 16° to 35° temperature, and immediately used. using a step of 0.02° and a scan rate of 0.039789°/min. The X’Pert Data Collector version 2.0 was used to analyze the Differential Scanning Calorimetry diffraction patterns. Crystallization and melting profiles of the pure compo- Hardness Measurements nents, mixtures, and solutions were determined in a DSC (model 2920; TA Instruments New Castle, DE, USA) Glass containers (2.4 cm 19 mm I.D.) were filled with × equipped with a refrigerated cooling unit. Prior to analysis, 3.0 g of the corresponding solution (90 °C) and stored the DSC was calibrated with indium (melting temperature at 25 °C for 24 h. We determined the penetration work of 156.6 °C and heat of melting of 28.45 J/g). An empty (gf*mm) of the corresponding gels using a texture ana- aluminum pan was used as a reference. The DSC cell was lyzer (LRFA 1500; Brookfield, USA) fitted with a plastic purged with nitrogen (99.99 % purity) flow of 25 mL/ cylindrical probe (35 mm length 12.7 mm diameter; × min. The sample (2.5–3 mg) sealed in the pan was heated TA10; Brookfield, USA). The measurements were obtained at 15 °C above the melting point of the compound in the at room temperature ( 25 °C) in compression mode, ≈ 1 3 J Am Oil Chem Soc Fig. 1 Heating thermograms for CW candelilla wax, C31 n-hentriac- Fig. 2 X-ray powder diffractograms for CW candelilla wax, C31 ontane, and C30 melissic acid. The insert is the enlarged section of n-hentriacontane, C30 melissic acid, and the C31/C30 mixture with a the heating thermogram between 40 and 60 °C for C30 0.2 mol fraction of C30 (x 0.2) 30 = penetrating 4 mm into the sample at 1 mm/s and return- pattern in the wide angle region (2θ > 20°) showed two ing to initial position at the same speed.
Details
-
File Typepdf
-
Upload Time-
-
Content LanguagesEnglish
-
Upload UserAnonymous/Not logged-in
-
File Pages8 Page
-
File Size-