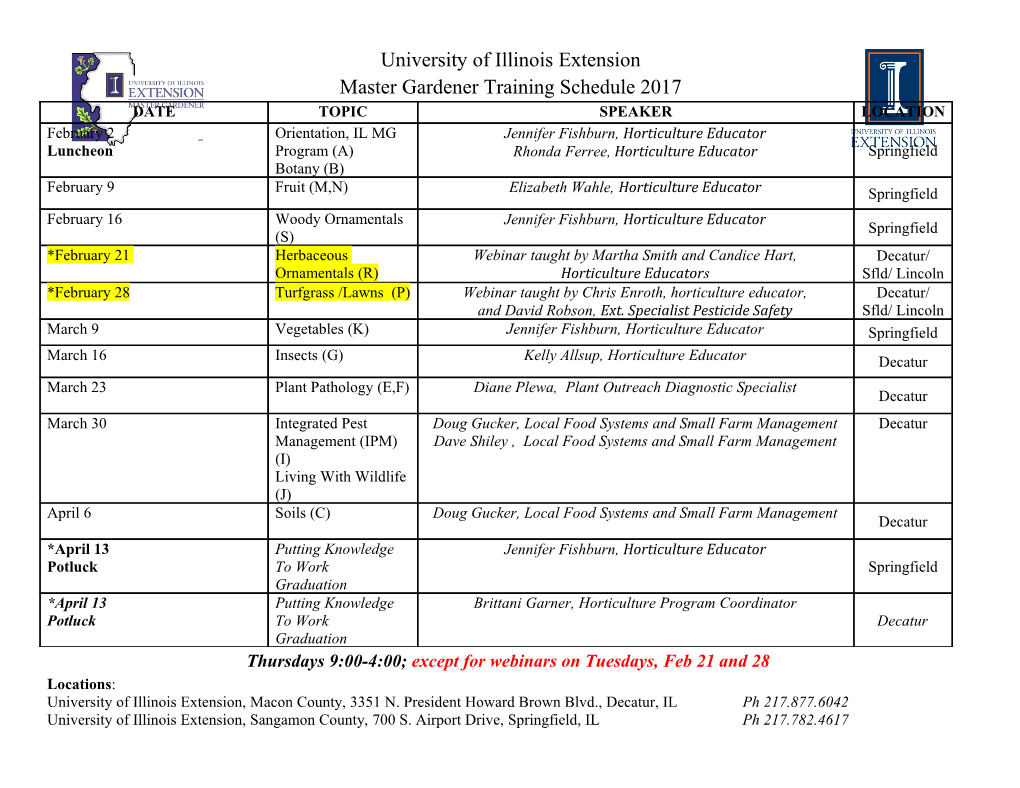
ARTICLES https://doi.org/10.1038/s41561-018-0297-z Early and persistent supershear rupture of the 2018 magnitude 7.5 Palu earthquake Han Bao 1, Jean-Paul Ampuero 2,3*, Lingsen Meng1, Eric J. Fielding 4, Cunren Liang3, Christopher W. D. Milliner4, Tian Feng1 and Hui Huang1 The speed at which an earthquake rupture propagates affects its energy balance and ground shaking impact. Dynamic mod- els of supershear earthquakes, which are faster than the speed of shear waves, often start at subshear speed and later run faster than Eshelby’s speed. Here we present robust evidence of an early and persistent supershear rupture at the sub-Eshelby speed of the 2018 magnitude 7.5 Palu, Indonesia, earthquake. Slowness-enhanced back-projection of teleseismic data provides a sharp image of the rupture process, along a path consistent with the surface rupture trace inferred by subpixel correlation of synthetic-aperture radar and satellite optical images. The rupture propagated at a sustained velocity of 4.1 km s–1 from its initiation to its end, despite large fault bends. The persistent supershear speed is further validated by seismological evidence of far-field Rayleigh Mach waves. The unusual features of this earthquake probe the connections between the rupture dynamics and fault structure. An early supershear transition could be promoted by fault roughness near the hypocentre. Steady rupture propagation at a speed unexpected in homogeneous media could result from the presence of a low-velocity damaged fault zone. lthough most earthquakes rupture at speeds lower than surface rupture on land where the east side moved north (positive in the shear wave velocity, faster, so-called supershear earth- Fig. 1) and the west side moved south. Pixel tracking of the optical quakes have been predicted by theory and simulations1,2 and images provides a complementary data set that resolves the two- A 3 observed in laboratory experiments and large strike-slip earth- dimensional (2D) horizontal deformation pattern (Methods and quakes4. Whether observable fault properties control the occur- Supplementary Fig. 10), where the north–south correlation maps rence of supershear rupture in nature is not completely understood. shows a similar result to the SAR pixel offset. Supershear ruptures have been proposed to occur on smooth and Both data sets indicate that the inferred surface rupture has straight faults5, and to be promoted on fault segments with well- major geometrical complexities and differences between the north- developed damage zones6,7 and on geometrically rough faults8. ern and southern portions (Fig. 1a and Supplementary Fig. 1). No On 28 September 2018, an earthquake with moment magnitude surface rupture was discerned north of the epicentre. The south- (Mw) 7.5 occurred in Sulawesi, Indonesia (Fig. 1). Its epicentre was ern end of the surface rupture is at 119.99° E, 1.47° S (label E on located about 80 km north of the city of Palu. The earthquake rup- Fig. 1a). The northern part of the rupture, from the epicentre to its tured along the Palu-Koro fault, a strike-slip left-lateral fault with a intersection with the Palu Bay coast at 119.83° E, 0.69° S (label B), geodetic slip rate of 42 mm yr–1 (ref. 9), a record of large earthquakes is less straight than the rupture from Palu city to the south. There with magnitudes from 7 to 8 (ref. 10) and previously identified seis- is a substantial right bend on the rupture at 119.83° E, 0.34° S (label mic hazard11. This event triggered a tsunami and landslides that A on Fig. 1a), over which the fault trace is offset by about 4 km in caused more than 2,000 casualties. the direction perpendicular to the main rupture strike. The rupture Here we focus on a feature of this earthquake that is impor- from Palu city (label C) to the south is very straight until it makes tant for our fundamental understanding of earthquake mechanics. a large left bend at 119.885° E, 1.185° S (label D on Fig. 1a). The left We present robust seismological evidence of an early and persis- bend is about 8.5 km along the diagonal and 6.5 km perpendicular tent supershear rupture that propagates steadily at a speed that is to the main fault strike. The northern right bend and southern left thought to be unstable. We further exploit remote sensing obser- bend are restraining and releasing, respectively, for the left-lateral vations of the rupture trace geometry to discuss possible relations Palu earthquake. The slip distribution measured from the optical between rupture speeds and fault structure. image correlation maps (Methods) reveals the variation of fault slip along the surface rupture. The slip profile shows a maximum slip of Remote sensing observations of the surface rupture 6 ± 0.5 m located near the city of Palu, and a clear difference in slip Analysis of synthetic aperture radar (SAR) and optical images pro- magnitude between the northern and southern segments, with an vides key constraints on the rupture geometry and the distribution average slip of 1.9 m and 4.7 m, respectively (Fig. 1b). of fault slip of the Palu earthquake. We measured the horizontal surface deformation due to the earthquake from a subpixel corre- Teleseismic back-projection supershear rupture imaging lation of the interferometric synthetic-aperture radar and optical A fast rupture during the Palu earthquake was first suggested by images (Methods). The SAR satellite tracks are very close to anti- a large ratio between rupture length (estimated from the distribu- parallel to the strike of the rupture, so the along-track displacements tion of aftershocks and from satellite images) and the rupture dura- are almost parallel to the fault strike and show the location of the tion inferred from teleseismic source time functions. Teleseismic 1Earth, Planetary and Space Sciences, University of California, Los Angeles, CA, USA. 2Université Côte d’Azur, IRD, CNRS, Observatoire de la Côte d’Azur, Géoazur, France. 3California Institute of Technology, Seismological Laboratory, Pasadena, CA, USA. 4Jet Propulsion Laboratory, California Institute of Technology, Pasadena, CA, USA. *e-mail: [email protected] 200 NatURE GEOSCIENCE | VOL 12 | MARCH 2019 | 200–205 | www.nature.com/naturegeoscience NATURE GEOSCIENCE ARTICLES abc Time (s) 01020 30 40 50 1 0.0° Po we r 0 0 0 HF radiator d A 0.5° S Strike 50 50 B Distance along str Palu Bay C Track Palu city 1.0° S ik 100 e 100 e (km) 30° N Distance from epicentre (km) D 0° 0 15 30 45 HF radiation time (s) 150 30° S 150 –5 05 1.5° S E 90° E 120° E 150° E Along-track displacement (m) 0 2 46 119.5° E 120.0° E Left-lateral fault slip (m) Fig. 1 | Surface rupture trace and supershear speed of the Palu earthquake. a, Along-track displacements from ALOS-2 SAR offsets (coloured image on land) and bathymetry (grey background offshore). The arrow labelled as ‘Track’ indicates the direction of measurement, –11.7°. The red star denotes the NEIC epicentre of the Palu earthquake. The green star is the relocated epicentre of the 28 September 2018 M 6.1 foreshock. The mainshock and foreshock focal mechanisms are also shown. The inferred surface rupture trace is indicated by a thin black line. Circles are the high-frequency (0.5~2 Hz) radiators imaged by the SEBP on data recorded by the Australia array, with size proportional to the relative energy and colour that represents the rupture time with respect to the mainshock origin time. b, Left-lateral slip distribution along the surface rupture measured from optical image correlation of the Sentinel-2 and Planet Labs data (Supplementary Fig. 10 gives the correlation maps). Fault slip is almost a factor of two larger on the southern segment through Palu city than north of the bay. c, Beam power as a function of time. Low-amplitude radiators after 45 s (grey) were not used in further analysis. d, Along-strike location and timing of radiators imaged by SEBP. Time is relative to the rupture origin time. Location is the horizontal position relative to the hypocentre, projected along the average strike direction (174°). The dashed line is a linear regression of the radiators close to the leading rupture front (circles with a blue rim are ignored). Error bars are location uncertainties derived from the slowness correction (Supplementary Fig. 11). e, The map shows the mainshock epicentre (red star) and stations of the Australia array used for SEBP (green triangles). source inversion properly constrains the rupture duration but suf- and by remote sensing. Coherent sources with significant beam fers from a strong trade-off between rupture size and rupture speed. power occur until approximately 45 s after the rupture initiation Owing to the advent of regional dense seismic arrays, teleseismic (Fig. 1c). This source duration is consistent with the half-duration of back-projection rupture imaging has become one of the essential 22.5 s reported by the routine United States Geological Survey techniques to constrain the kinematic rupture properties of large (USGS) W-phase analysis (as in Data availability). The high-fre- earthquakes, such as rupture lengths, directions, speeds and seg- quency sources follow an overall linear rupture path towards South- mentation12. Without prior knowledge of the fault geometry or the South-East, consistent with the surface fault traces identified by our rupture speed, back-projection determines the location, timing and SAR analysis (Fig. 1a). In two separate occasions, at around 10 s and relative power of coherent high-frequency sources by exploiting 25 s, we observe more dispersed radiators, which suggests higher rup- the coherency of seismic waveforms across dense arrays.
Details
-
File Typepdf
-
Upload Time-
-
Content LanguagesEnglish
-
Upload UserAnonymous/Not logged-in
-
File Pages8 Page
-
File Size-