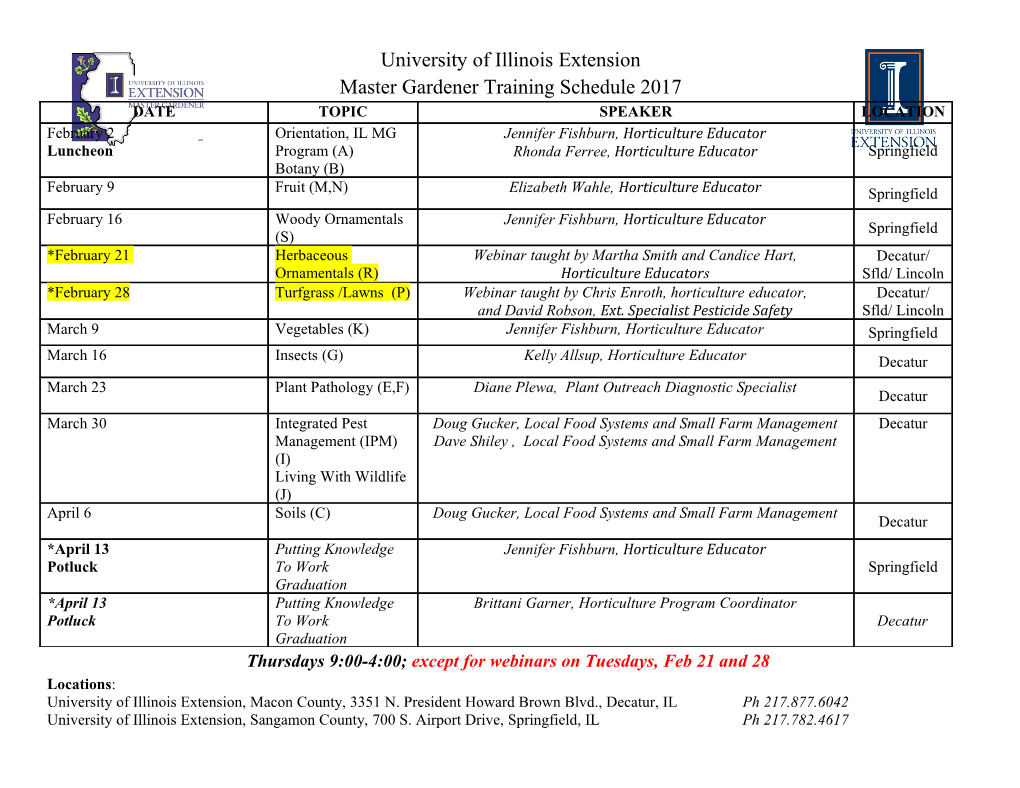
The Elusive 5′-Deoxyadenosyl Radical: Captured and Characterized by EPR and ENDOR Spectroscopies. Hao Yang1, Elizabeth C. McDaniel2, Stella Impano2, Amanda S. Byer2, Richard J. Jodts1, Kenichi Yokoyama3, William E. Broderick2, Joan B. Broderick2*, Brian M. Hoffman1*. 1Department of Chemistry, Northwestern University, Evanston, IL 60208. 2Department of Chemistry & Bio- chemistry, Montana State University, Bozeman, MT. 59717. 3Department of Biochemistry, Duke University, Durham, NC. Supporting Information Placeholder ABSTRACT: The 5¢-deoxyadenosyl radical (5¢-dAdo•) cofactor. We now know that coenzyme B12 abstracts a substrate H-atom as the first step in rad- serves as a reversible radical generator, un- ical-based transformations catalyzed by adeno- dergoing Co-C bond homolysis to give a 5’- sylcobalamin-dependent and radical S-adenosyl-L- deoxyadenosyl radical (5¢-dAdo•) that initi- methionine (RS) enzymes. Notwithstanding its cen- ¢ ates chemistry by H-atom abstraction from tral biological role, 5 -dAdo• has eluded character- 3 ization despite efforts spanning more than a half- substrate. The involvement of 5¢-dAdo• has century. Here we report generation of 5¢-dAdo• in been shown primarily through label-transfer a RS enzyme active site at 12 K, using a novel ap- studies, for example by observing incorpora- proach involving cryogenic photoinduced electron tion of substrate deuterons into adenosylco- + transfer from the [4Fe-4S] cluster to the coordi- balamin during catalysis.4-5 Important in- nated S-adenosylmethionine (SAM) to induce ho- sights into its behavior have been attained molytic S-C5¢ bond cleavage. We unequivocally re- through kinetic and spectroscopic investiga- veal the structure of this long-sought radical spe- cies through use of electron paramagnetic reso- tions in which the Co-C5¢ bond of B12 is pho- nance (EPR) and electron nuclear double reso- tolytically cleaved, after which the 5¢-dAdo• nance (ENDOR) spectroscopies with isotopic label- radical can either reform that bond or pro- ing, complemented by density-functional computa- ceed to react with substrate.6-7 However, tions: a planar C5¢ (2pπ) radical (~70% spin occu- strenuous efforts over many years8 to trap pancy); the C5¢(H)2 plane is rotated by ~ 39° relative and fully characterize the 5¢-dAdo• formed to the C5¢-C4¢-(C4¢-H) plane, placing a C5¢-H anti- from B12 have been unavailing: the radical has periplanar to the ribose-ring oxygen, which helps stabilize the radical against elimination of the 4¢-H. simply been too reactive to trap. The significance of the 5¢-dAdo• radical in biology broadened dramatically with the The discovery by Barker in 1958 of a cobal- recognition that S-adenosylmethionine amin involved in catalyzing an isomerization (SAM) plays a role analogous to that of coen- 1 9-10 reaction, and its subsequent identification zyme B12 in initiating radical reactions. as adenosylcobalamin (coenzyme B12 (Fig 1)) This role for SAM was first suggested for ly- 11-12 by Hodgkin in 1961,2 set the stage for decades sine 2,3-aminomutase and pyruvate for- 13-14 of study focused on the biochemical reac- mate-lyase activating enzyme, but it is now tions of this remarkable organometallic implicated throughout the vast and diverse RS superfamily.15 Until recently, the accepted Fig. 1. Adenosylcobalamin/coenzyme B12 (left) and S-adenosylmethionine bound to a [4Fe-4S] cluster in the active site of RS enzymes (modeled at right) both serve as precursors to the 5¢-dAdo• radical intermediate (center). mechanism for the RS enzymes involved the Co(II; S = ½) corrinoid and the S= ½ rad- SAM coordination to the unique iron of the ical result in a broad, poorly-defined elec- [4Fe-4S] cluster in the active site; electron tron paramagnetic resonance (EPR) signal transfer from the reduced [4Fe-4S]+ cluster to that is not amenable to analysis.21 A sharp SAM promoted reductive cleavage of SAM to fluid-solution FT-EPR spectrum acquired give methionine and 5¢-dAdo•, which initi- upon photolysis of B12 and thought to arise ates chemistry via substrate H-atom abstrac- from 5¢-dAdo•, is instead the result of rapid tion.15-16 The recent discovery of the catalyti- radical rearrangement rather than 5¢-dAdo• cally competent organometallic intermediate itself (see SI Text, Fig. S2).22 An organic rad- W formed during the RS reaction provides a ical that accumulates during reaction of the new twist on the mechanism, by implicating RS enzyme spore photoproduct lyase with a coenzyme B12-like organometallic interme- SAM at 40 ˚C also has been proposed to be diate with an Fe-C5¢ bond that undergoes ho- 5¢-dAdo•,23 but we show in the SI that this molytic bond cleavage to release 5¢-dAdo•.16- radical too is not 5¢-dAdo• (see SI Text, Fig 18 S3, Table S1). Insights into the characteristics of the 5¢- Here, after more than half a century of ef- dAdo• radical intermediate in RS enzymes forts by numerous investigators to trap and have been achieved via the SAM analog 3¢,4¢- characterize the biologically central 5¢- anhydro-S-adenosylmethionine (anSAM), dAdo• radical, we demonstrate the for- which generates an allylically stabilized 5¢- mation of 5¢-dAdo• in a RS enzyme active dAdo• analog that is amenable to character- site, by using a novel approach involving ization,19-20 but so far attempts to generate photoinitiated electron transfer from the re- and characterize the 5¢-dAdo• radical itself duced [4Fe-4S]+ cluster to the coordinated have not been productive. For example, pho- SAM. The resulting 5¢-dAdo• is definitively tolysis of coenzyme B12 appears to produce 5¢- identified through the use of isotopically la- dAdo•, but spin-spin interactions between beled SAM combined with EPR and electron-nuclear double resonance Photolysis was carried out in situ using a 450 (ENDOR) spectroscopy, with its structure an- nm Thorlabs diode laser. The time course of alyzed using density functional theory (DFT) 12 K photolysis of (PFL-AE1++SAM) complex computation. was monitored during intra-cavity photolysis in an X-band EPR spectrometer. The in situ Experimental Methods photolysis of the (PFL-AE1++SAM) complex Materials pre-reduced to the [4Fe-4S]+ state using ei- 13 15 [Methyl- C]-L-methionine, N-L-methio- ther deazariboflavin or dithionite gives iden- nine, and 2,8-D2-1¢,2¢,3¢,4¢,5¢,5¢¢-D6-adenosine tical EPR spectra, Figure S1. The in situ pho- 5¢-triphosphate salt solution were purchased tolyzed samples were subjected to X- and Q- from Cambridge Isotope Laboratories, Inc. band CW EPR and Q-band ENDOR meas- 13 15 [ C10, N5]-adenosine 5¢-triphosphate sodium urements as described below. salt solution was purchased from Sigma and EPR and ENDOR Measurements 3,3,4,4-d4-L-methionine was obtained from X-band CW EPR spectroscopy was con- CDN Isotopes. ducted on a Bruker ESP 300 spectrometer Protein and SAM Preparation equipped with an Oxford Instruments ESR PFL-AE, unlabeled SAM, and labeled 910, while Q-band CW EPR spectroscopy SAMs were prepared as previously re- was conducted on a Bruker EMX spectrom- 24 ported. eter equipped with an Oxford Instruments Photolysis Mercury iTC continuous helium flow cryo- Pyruvate formate-lyase (PFL-AE) was pre- stat. Typical experimental parameters were at pared for photolysis by reducing the enzyme 12 K and 40 K, 9.38 GHz or 34.0 GHz, and 10 and adding labeled or unlabeled SAM. Pho- G modulation amplitude. EPR simulations 5.2.23 toreduced PFL-AE was prepared as previ- were performed with the EasySpin pro- 25 ously described24 with minor alterations. gram operating in Matlab. PFL-AE (0.55 mM), dithiothreitol (DTT, 1.0 35 GHz CW and pulse ENDOR spectro- mM) and deazariboflavin (200 µM, dissolved scopic data were collected on spectrometers, in DMSO) were combined in buffer (50 mM described previously,26-28 that are equipped Tris, 100 mM KCl, pH 7.5) in an anaerobic with liquid helium immersion dewars for COY chamber. The sample was irradiated by measurements at 2 K. The CW measure- a 500 W halogen lamp for 1 h while the sam- ments employed 100 kHz field modulation ples remained in an ice water bath. Labeled and dispersion-mode detection under rapid or unlabeled SAM (5.5 mM) was then added. passage conditions. 1H CW ENDOR spectra The samples were then transferred to EPR employed bandwidth broadening of the RF tubes and frozen in liquid nitrogen inside to 100 kHz to improve signal-to-noise.29 1H the COY chamber. CW ENDOR spectra were collected using Dithionite reductions were performed sim- the stochastic-field modulation detected 30 ilarly, as follows: PFL-AE (0.55 mM), DTT (1.0 ENDOR sequence, to improve ENDOR line mM), and sodium dithionite (3.0 mM) were shape. For a single molecular orientation and 1 13 combined in an anaerobic COY chamber for nuclei with nuclear spin of I = /2 ( C), the 1 and allowed to sit 3 min before SAM (5.5 mM) ENDOR transitions for the ms = ± /2 electron was added. The samples were flash frozen as manifolds are observed, to first order as described above and stored in liquid nitro- shown in equation: gen. A intermediate in RS enzymes could be uu=± (eq. 1) ± n 2 cleaved photolytically, similar to the Co-C5¢ bond of coenzyme-B12. If this were to occur, where un is the nuclear Larmor frequency, and A is the orientation-dependent hyper- fine coupling. When un > A/2, the pattern is a doublet split by A and centered at un; when un < A/2, the pattern is a doublet centered at A/2 and split by 2un. DFT calculations All DFT computations of 5′-dAdo• were performed in ORCA 4.0.1.31 The initial coor- dinates for the 5¢-dAdo fragment were taken + from a 1.5 Å resolution crystal structure of a Fig.
Details
-
File Typepdf
-
Upload Time-
-
Content LanguagesEnglish
-
Upload UserAnonymous/Not logged-in
-
File Pages13 Page
-
File Size-