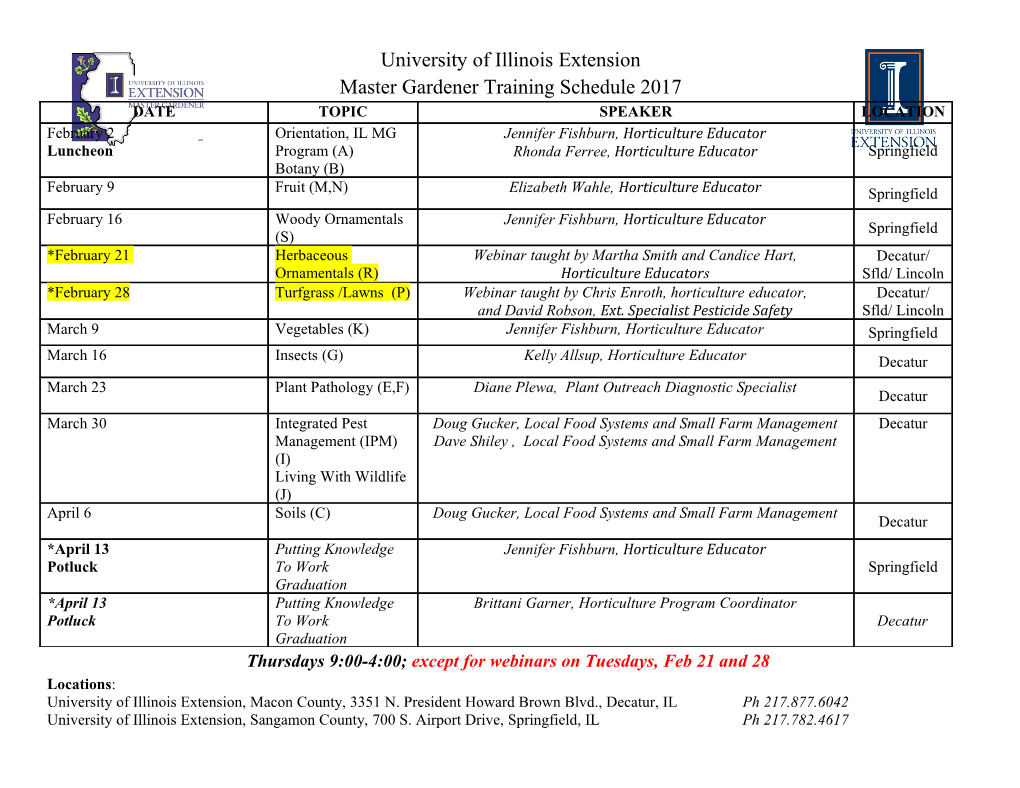
JOURNAL OF MATERIALS SCIENCE 28 (19931 3693-3708 Review Thermite reactions: their utilization in the synthesis and processing of materials L. L. WANG, Z. A. MUNIR, Y. M. MAXIMOV* Department of Mechanical Aeronautical, and Materials Engineering, University of Cafifornia, Davis, CA 95616, USA A class of exothermic reactions between, typically, a metal and an oxide, commonly referred to as thermite reactions, is reviewed with emphasis on their utilization in the synthesis and processing of materials. Theoretical and experimental results relating to ignition and combustion (self-propagation) characteristics of these reactions are presented. 1. Introduction actions have become important in the synthesis of The word "thermit" was first coined by Goldschmidt refractory ceramic and composite materials [38-57], in 1908 [1] to describe exothermic reactions involving and in the preparation of ceramic linings in metallic reduction of metallic oxides with aluminium to form pipes [58-70]. There are also many other materials- aluminium oxide and metals or alloys. This type of related processing and heat-generation inventions reaction is characterized by a large heat release which which utilize thermite reactions [71-85]. While most is often sufficient to heat the product phases above of the focus is on the beneficial aspect of thermite their melting points. For example, the thermite reac- reactions, accidental explosion in chemical plants and tion 2AI + Fe203 --* 2Fe + A120 3 can attain a tem- mines involving such reactions are of great safety perature higher than 3000~ which is above the concern [86, 87]. This brief review demonstrates the melting points of both iron and A1203. Currently, the importance of this class of industrial reactions. We term thermite reaction is used to describe a much provide a review of the theoretical and experimental broader class of reactions and can be defined as an work relating to thermite reactions and discuss the exothermic reaction which involves a metal reacting important examples of their utilizations. with a metallic or a non-metallic oxide to form a more stable oxide and the corresponding metal or non- metal of the reactant oxide. This is a form of oxida- 2. Thermodynamic considerations tion-reduction reaction which can be written in a As indicated above, thermite reactions are oxida- general form as tion-reduction processes. There are a number of fac- tors involved in the selection of a reducing agent for M + AO--, MO + A + AH (1) a particular oxide. The tendency for a metal to reduce where M is a metal or an alloy and A is either a metal an oxide depends, of course, on the free energy of or a non-metal, MO and AO are their corresponding formation of its oxide. Fig. 1 presents the Gibbs free oxides, and AH is the heat generated by the reaction. energy of formation as a function of temperature for Because of the large exothermic heat, a thermite reac- a series of possible reducing metals (A1, Mg, Ca, Zr, Zn tion can generally be initiated locally and can become and Ti) and several other common reducing agent s (Si, self-sustaining, a feature which makes their use ex- C and H2) [88, 89]. All the reactant metals show tremely energy efficient. The fact that many thermite negative Gibbs free energies of oxide formation over reactions yield a molten product that consists of a wide temperature range. Most of the metals have a heavier metallic phase and a lighter oxide phase higher reducing tendency than the non-metal reducing which can be separated by gravity, makes these reac- agents, and the extent of their reducing tendency de- tions potentially useful in a variety of metallurgical creases as temperature increases. Both calcium and applications [2-20]. Because the self-sustaining nature magnesium have higher Gibbs free energies of oxide of thermite reactions can be adjusted by the addition formation at low temperatures, but their reducing of an inert diluent, they are often used as experimental tendency decreases more sharply at elevated temper- models for solid combustion studies [21-35] and for atures. In addition, both calcium and magnesium are pyrotechnic uses [36, 37]. More recently, thermite re- more volatile, boiling at 1757 and 1363 K, respectively * Visiting scientist. Permanent address: Tomsk Branch of the Institute for Structural Macrokinetics. Russian Academy of Sciences, Tomsk, Russia 0022-2461 1993 Chapman & Hall 3693 & ] [ ~.J_.........-~ TiO2 -1oo / j.. Nb O, t zO3 "~ " ~ [ ~Fe203 _ _ L ] ~ NiO~-- I }iii 00, oo,o I 0 1000 2000 3000 -160, I I Temperature (K) 0 1000 2000 3000 Temperature (K) Figure I Free energy of formation for oxides. Figure 2 Free energy of thermite reactions with aluminium as the reducing agent. (at 1 arm pressure), and thus are less desirable for adiabatic temperature which can be calculated from certain applications because of high reaction pressure the enthalpy of the reaction and the heat capacity of and vaporization losses [8]. Both aluminium and the product phases with the assumption of adiabatic zirconium have comparable reducing tendency, but conditions. A large number of oxides can be reduced aluminium is more commonly used, because it is more by aluminium up to relatively high temperatures readily available. Its physical properties and those of (2500 K), as shown in Fig. 2. These aluminium-based its oxides are more suitable for the requirements of thermite reactions result in production of A1203 and several metallurgical applications [5], as will be dis- the elemental components corresponding to the reac- cussed later. tant oxides. Using the usual thermodynamic approach Another factor is the consideration of the exother- [90, 91], the adiabatic temperatures, Tad, for these micity of the thermite reactions. The heat released thermite reactions were calculated and are presented from the reaction heats up the product to the in Table I. In many cases, the adiabatic temperature TAB L E I Adiabatic combustion temperatures and melting points of the product metals Reaction Ta(K) a Tmp of metal (K) b I. Formation of common structural metals A1 + 1/2Fe203 --, Fe + 1/2A1203 3622 1809 AI + 3/2NIO ---,3/2Ni + 1/2A12Oa 3524 1726 A1 + 3/4TIO2 --* 3/4Ti + 1/2A1203 1799 1943 A1 + 3/8Co304 ~ 9/8Co 4- 1/2A1203 4181 1495 II. Formation of refractory metals ' AI + 1/2Cr203 --* Cr + 1/2A1203 2381 2130 A1 + 3/10VaOs ~ 6/10V + 1/2A1203 3785 2175 AI + 3/10TaaOs ~ 6/10Ta + 1/2A1203 2470 3287 A1 + 1/2MOO3 ---, l/2Mo + 1/2A1203 4281 2890 A1 + 1/2WO3 ~ 1/2W + 1/2A120a 4280 3680 A1 + 3/10Nb2Os --* 6/10Nb + 1/2A1203 2756 2740 III. Formation of other metals and non-metals AI + 1/2B203 ~ B + 1/2AlzO3 2315 2360 AI + 3/4PBO2 --, 3/4Pb + 1/2A1203 > 4000 600 A1 + 3/4MNO2 ~ 3/4Mn + 1/2A1203 4178 t517 A1 + 3/4SIO2 ~ 3/4Si + 1/2AIzO3 1760 1685 IV. Formation of nuclear metals AI + 3/-16U308 --+ 9/16U + 1/2A1203 2135 1405 A1 + 3/4PuO/--* 3/4Pu + 1/2A1203 796 913 The calculation of T,~ddoes not take into account vaporization of the product phases. b Tmp of A1203 is 2315 K. 3694 exceeds both melting points of the product phases. play a significant role in initiating the combustion These include reactions that reduce Fe203, NiO, reaction. For example, the ignition of the CrO3-A1 C0304, Cr203, V203, MoOa, WO3, PbO2, MnO/, mixture can occur at as low a temperature as about and Nb2Os, while the reaction to reduce B203 170~ which is the decomposition temperature of reaches the melting point of A1203. Only in a few CrO3. Finally, with the Class 4 oxides, further oxida- cases (e.g. reduction of TiO2 and PuOz by aluminium) tion takes place in air, and the heat liberated from this is the adiabatic temperature below the melting point reaction can heat the specimen to the ignition point of of both product phases. The adiabatic temperature the thermite reaction. An example of such a case is the provides not only a quantitative measure of the ignition of FeO-A1 in air, which is initiated by the exothermicity of the reaction, but also a quick deter- reaction of FeO with oxygen to form a higher oxide. mination of the propensity of the reaction to self- The initiation of thermite reactions can be accomp- propagate. In self-propagation, the reaction proceeds lished in a variety of methods. They can be ignited by in a combustion form in which the heat generated a combustion wave from a chemical reaction (or ig- from the locally ignited region can subsequently trig- niter) [21-25], an electrical current [94, 95], radiation ger the reaction in the adjacent reactant layer, thus the energy from a heat source or a laser beam [96, 97], or reaction zone moves in the form of a wave until all the by mechanical impact [86, 87]. The ignition process of reactants are consumed. As a rule, the reaction can a thermite reaction by a combustion wave has been self-propagate if the Taa exceeds 2000 K [91]. More- most intensely studied, both theoretically and experi- over, the information of T~a also provides some insight mentally [21-24]. The investigation of this ignition to the possible states of interaction, whether in solid, process is not only of great interest as a theoretical liquid, gas, or a combination of these. The high reac- problem of the interaction between two reactive sys- tion temperatures of thermite systems ensure the pos- tems, but also for a number of practical applications, sibility of achieving equilibrium conditions. It is, such as in the choice of optimum composition of the therefore, suitable to use thermodynamic calculations igniter for pyrotechnical uses [36, 37]. based on minimization of the total Gibbs free energy The electrical energy required to ignite thermite of the system to obtain equilibrium distribution of mixtures has been investigated [94].
Details
-
File Typepdf
-
Upload Time-
-
Content LanguagesEnglish
-
Upload UserAnonymous/Not logged-in
-
File Pages16 Page
-
File Size-