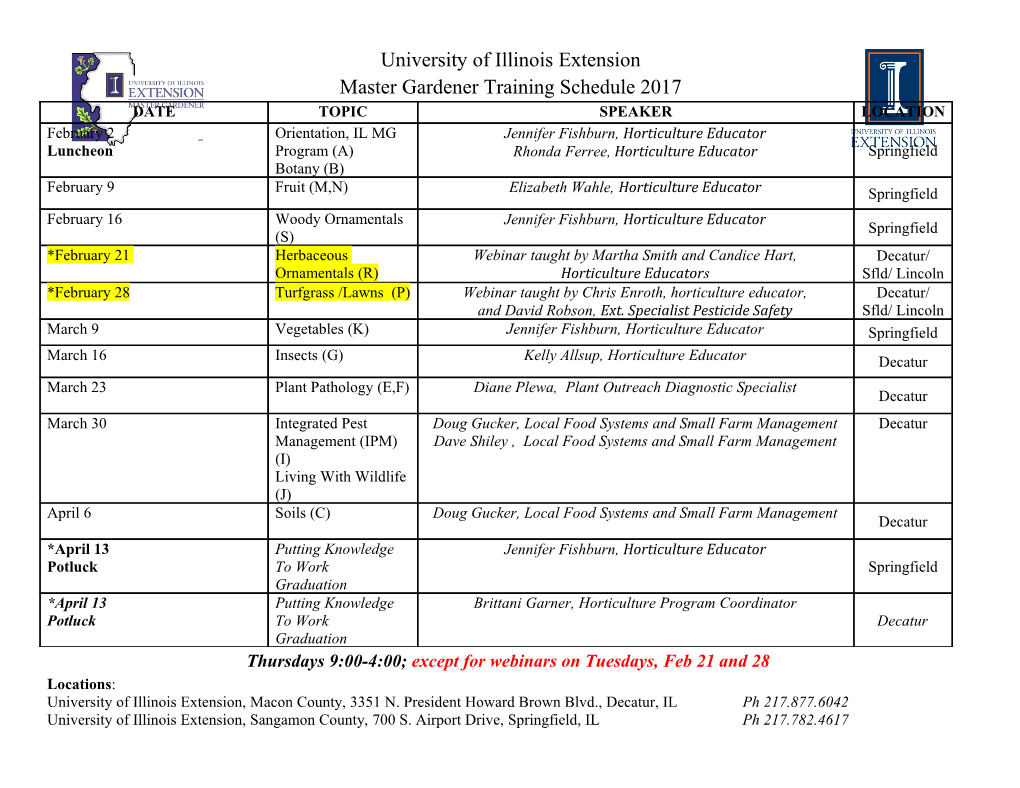
This is the submitted version of the following article: Zuo Y., Xu X., Zhang C., Li J., Du R., Wang X., Han X., Arbiol J., Llorca J., Liu J., Cabot A.. SnS2/g-C3N4/graphite nanocomposites as durable lithium-ion battery anode with high pseudocapacitance contribution. Electrochimica Acta, (2020). 349. 136369: - . 10.1016/j.electacta.2020.136369, which has been published in final form at https://dx.doi.org/10.1016/j.electacta.2020.136369 © https://dx.doi.org/10.1016/j.electacta.2020.136369. This manuscript version is made available under the CC-BY-NC-ND 4.0 license http://creativecommons.org/licenses/by-nc-nd/4.0/ SnS2/g-C3N4/Graphite Nanocomposites as Durable Lithium-Ion Battery Anode with High Pseudocapacitance Contribution Yong Zuo,+,a,b Xijun Xu,+,c,d Chaoqi Zhang,a,b Junshan Li,a,b Ruifeng Du,a,b Xiang Wang,a,b Xu Han,e Jordi Arbiol,e,f Jordi Llorca,g Jun Liu,*,c,d Andreu Cabot *,a, f a Catalonia Institute for Energy Research – IREC, Sant Adrià de Besòs, Barcelona, 08930 (Spain) b Departament d’Enginyeria Electrònica i Biomèdica, Universitat de Barcelona 08028 Barcelona (Spain) c Guangdong Provincial Key Laboratory of Advanced Energy Storage Materials, School of Materials Science and Engineering, South China University of Technology, Guangzhou 510641 (P. R. China) d State Key Laboratory of Pulp and Paper Engineering, South China University of Technology, Guangzhou 510641 (P. R. China) e Catalan Institute of Nanoscience and Nanotechnology (ICN2), CSIC and BIST, Campus UAB, Bellaterra, 08193 Barcelona (Spain) f ICREA, Pg. Lluís Companys 23, 08010 Barcelona (Spain) g Institute of Energy Technologies, Department of Chemical Engineering and Barcelona Research Center in Multiscale Science and Engineering, Universitat Polittècnica de Catalunya, EEBE, 08019 Barcelona (Spain) + These authors contributed equally to this work. Abstract: Tin disulfide is a promising anode material for Li-ion batteries (LIB) owing to its high theoretical capacity and the abundance of its composing elements. However, bare SnS2 suffers from low electrical conductivity, which results in poor rate performance and cycling stability. Herein, we present a solution-based strategy to grow SnS2 nanostructures within a matrix of porous g-C3N4 (CN) and high electrical conductivity graphite plates (GPs). We test the resulting nanocomposite as anode in LIBs. First, SnS2 nanostructures with different geometries are tested, to find out that thin SnS2 nanoplates (SnS2-NPLs) provide the highest performances. Such SnS2-NPLs, incorporated into hierarchical SnS2/CN/GP nanocomposites, display excellent rate capabilities (536.5 mAh g-1 at 2.0 A g-1) and an outstanding stability (~99.7 % retention after 400 cycles), which are partially associated with a high pseudocapacitance contribution (88.8 % at 1.0 mV s-1). The excellent electrochemical properties of these nanocomposites are ascribed to the synergy created between the three nanocomposite components: i) thin SnS2-NPLs provide a large surface for rapid Li-ion intercalation and a proper geometry to stand volume expansions during lithiation/delithiation cycles; ii) porous CN prevents SnS2-NPLs aggregation, habilitates efficient channels for Li-ion diffusion and buffer stresses associated to SnS2 volume changes; and iii) conductive GPs allow an efficient charge transport. 1 Keywords: Nanocomposite, Li-ion battery, anode, tin disulfide, pseudocapacitance 2 1. Introduction Lithium-ion batteries (LIBs) are widely commercialized for portable applications owing to their notable volumetric energy density and their flexible and lightweight design. However, commercial devices suffer from low stability, and their potential energy densities and charging rates are far from those potentially attained by LIBs, which leaves plenty of room for improvement of their design and components, particularly of their anode materials.[1–3] Commercial LIBs use graphite anodes, which provide a modest theoretical energy density, 372 mAh g−1,[4,5] and are far away from the requirements of the next generation of high-energy and high-power LIBs.[6] Therefore, alternative anodes based on Si,[7,8] Ge [9,10] or Sn[11–13] are being actively developed. + Among the different materials able to intercalate or react with Li , sulfides and particularly SnS2 are especially interesting due to the abundance of its elements and the ability to simultaneously interact with + Li through two different bulk mechanisms: Li-S reaction and Sn-Li alloying. SnS2 anodes have a -1 + - potential capacity of 1231 mAh g ascribed to the combination of the Li-S reaction (SnS2 + 4Li + 4e → −1 Sn + 2Li2S, 586 mAh g capacity contribution)[14] and the Sn-Li alloying/dealloying reaction (Sn + + - −1 4.4Li + 4.4e → Li4.4Sn, 645 mAh g capacity contribution).[15,16] Besides, oxides and chalcogenides may provide a significant additional energy storage capacity contribution in the form of a rapid surface- related pseudocapacity.[3,17,18] SnS2 displays a two-dimensional (2D) hexagonal structure with layers of tin atoms sandwiched between two close-packed layers of sulfur atoms. Adjacent sandwiches, separated a distance of 0.59 nm, are hold together by Van der Waals forces.[19–21] This layered structure allows for the effective and fast + diffusion of Li (0.076 nm) to interact with Sn and S. However, during charge/discharge processes, SnS2 suffers from huge volumetric changes in large part related to the expansion/contraction in the c direction, i.e. across the planes, its softer crystallographic direction. This volumetric changes may result in the pulverization and detachment of the material from the current collector.[22–24] Additionally, the low electrical conductivity of SnS2 provides a poor rate performance. These limitations lead to a deficient cyclability, especially at high current densities.[25–28] To overcome its flaws as anode material in LIBs, SnS2 needs to be nanostructured[29–32] and combined with high surface area materials, e.g. carbon-based networks, to improve dispersion and simultaneously provide the necessary paths for electrolyte diffusion and electron transport.[33–35] Graphene is considered as one of the best materials for these last roles,[25,36] owing to its large surface, extraordinary electrical conductivity, and convenient flexibility that allows accommodating the conductive network to the volume expansion of the active material.[37] Graphene can interact with Li+ itself, delivering additional storage capacity. To maximize this interaction, graphene can be doped with large concentrations of N atoms which interact more efficiently with Li+ due to the higher 3 electronegativity of nitrogen compared with carbon.[38–40] In this direction, graphitic carbon nitride (g- C3N4, CN) is regarded as the analogue to graphite with the highest level of nitrogen, and thus may provide a significant Li+ storage capacity. [6,41,42] CN also has a 2D layered structure in which p-conjugated graphitic planes are composed by alternate arrangements of sp2 hybridized carbon and nitrogen atoms.[43,44] Advantageously, CN can be easily produced in a scalable way by the thermal decomposition of urea at moderate temperatures.[45,46] However, such CN is usually characterized by a low electrical conductivity due to an intrinsic porous microstructure.[41,47] Thus a highly conductive material needs to be additionally introduced to provide the necessary paths for electron transport. Herein, we combine SnS2 nanostructures with high surface area and porous CN and high electrical conductivity graphite plates (GPs) in hierarchical nanocomposites, and analyze their performance as anode material in LIBs. We initially identify the SnS2 nanostructure geometry providing better electrochemical performance by producing and testing SnS2 particles with different shapes. The better performing SnS2 nanostructure is then combined with porous CN, which we demonstrate is able to improve to some extent the electrode cycling stability. Finally, we analyze the effect of additionally incorporating conductive GP into the mixture to further improve performance. 4 2. Experimental section . Chemicals: Tin(IV) chloride pentahydrate (SnCl4 5H2O, 98%), sulfur powder (99.8%), tetrahydrofuran (THF, ≥99%), and GP (99 % carbon basis, -325 mesh) were purchased from Sigma-Aldrich. 1-octadecene (ODE, 90%) was purchased from Alfa-Aesar. Oleylamine (OAm, 80-90%), oleic acid (OAc, 70%), and urea (99 %) were purchased from Acros. Super P, polyvinylidene fluoride (PVDF) and porous polypropylene film (Celgard2400) were purchased from Shenzhen Kejing Star Technology Co. LTD. N- methy1-2-pyrrolidone (NMP, 99%), ethylene carbonate (EC), diethylcarbonate (DEC) and fluoro ethylenecarbonate (FEC) were purchased from Aladdin. Toluene, hexane, isopropanol and ethanol were of analytical grade and obtained from various sources. GPs were cleaned using diluted HCl and ethanol under sonication. All other chemicals were used as received, without additional purification. Aqueous solutions were prepared using Milli-Q water (DW, 18.2 MΩ.cm). Synthesis of SnS2 nanostructures and SnS2/CN and SnS2/CN/GP nanocomposites: Plate- and flower- shaped SnS2 nanostructures were produced following our recently reported protocol.[21] Briefly, to . prepare SnS2 nanoplates (SnS2-NPLs), SnCl4 5H2O (2 mmol), ODE (16 mL), OAc (4 mL), OAm (2 mL) and THF (1 mL) were added into a three-neck round flask (50 mL). The solution was stirred using a magnetic stirring bar and a syringe was inserted into the solution to bubble Ar. The solution was then heated up to 140 °C and kept at this
Details
-
File Typepdf
-
Upload Time-
-
Content LanguagesEnglish
-
Upload UserAnonymous/Not logged-in
-
File Pages26 Page
-
File Size-