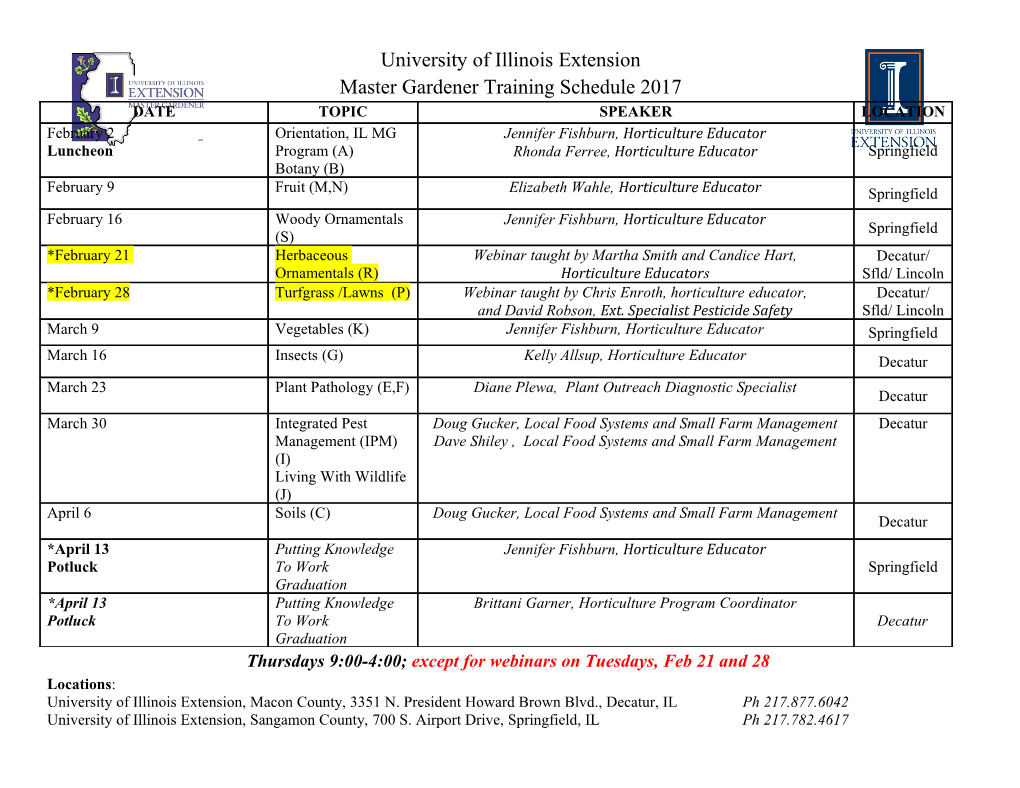
LA-10500-MS LA— 10500-MS UC-20d DES6 001238 Issued: August 1985 Compact Reversed-Field Pinch Reactors (CRFPR): Fusion-Power-Core Integration Study C. Copenhaver R. A. Krakowski N. M. Schnurr R. L. Miller C. G. Bathke • R. L Hagenson* C. R. Mynard A. D. Chaffee C. Cappiello J.W.Davidson •Consultant at Los Alamos. Phillips Petroieun? Company, Bartlesville, OK 74004. Los Alamos National Laboratory Los Aîamos,New Mexico 87545 m: n OF THIS WBWR S ^L TABLE OF CONTENTS 1. INTRODUCTION 2 1.1. Background ..... ... 2 1.2. Scope 5 1.3. Fusion-Power-Core (FPC) Configuration and Standard Conditions . 6 2. FUSION-POWER-CORE MODELS 15 2.1. Two-Dimensional Neutronics 15 2.2. FPC Thermal-Hydraulics Model 22 2.2.1. First-Wall/Second-Wall Models 24 2.2.1.1. First-Wall Structural Analysis .... 26 2.2.1.2. First-Wall Thermal-Hydraulic Analysis 27 2.2.2. Limiter 31 2.2.3. Blanket 35 2.2.3.1. Blanket Hydraulic Analysis 35 2.2.3.2. Blanket Thermal Analysis 39 2.2.3.3. Channel Design 42 3. FUSION-POWER-CORE INTEGRATION 47 3.1. Power-Plant Energy Balance . « 47 3.2. Thermal-Hydraulic Design 49 3.2.1. Limiter Analysis 53 3.2.2. First-Wall/Second-Wall Analysis 54 3.2.3. Blanket Analysis 56 3.2.4. Shield Analysis 61 3.2.5. Design Summary ....... 62 3.3. Mechanical Design . 68 3.4. Base-Case Design Summary ... 72 3.5. Derated FPC Design Options id Tradeoffs 72 4. FPC MAINTENANCE APPROACH 81 4.1. Plant Layout Options 81 4.2. Plant Subsystems 90 4.2.1. PbLi Pumps 90 4.2.2. PbLi Superheater, Steam Generator, Economizer 90 4.2.3. Tritium Removal . 91 4.2.4. Special Maintenance Tools and Disconnects 92 4.3. FPC Replacement Procedures 96 4.3.1. Vertical Replacement 96 4.3.2. Horizontal Replacement 97 5. AFTERHEAT AND RADIOACTIVITY 101 5.1. Afterheat Model 102 5.1.1. Neutronics Model 102 5.1.2. Thermal-Hydraulic Model 104 5.1.3. Natural-Circulation Model 109 5.2. Afterheat Results , 113 5.3. Emergency Cooling System „ . 122 5.3.1. General Description 122 5.3.2. ECS Description for CRFPR (20) Design 123 5.3.3. ECS Component Description 126 5.3.3.1. Accumulators 126 5.3.3.2. Pumps 126 5.3.3.3. Residual Heat Exchangers 127 iv COMPACT REVERSSD-FIELD PINCH REACTORS (CRFPR): FUSION-POWER-CORE INTEGRATION STUDY by C. Copenhaver, R. A. Krakowski, N. M. Schnurr, R. L. Miller, C. G. 3athke, R. L. Hagenson, C. R. Mynard, A. D. Chaffee, C. Cappiello, J. W. Davidson ABSTRACT Using detailed two-dimensional neutronics studies based on the results of a previous framework study (LA-10200-MS), the fusion-power-core (FPC) integration, maintenance, and radio-activity/afterheat control are examined for the Compact Reversed-Field Pinch Reactor (CRFPR). While maintaining as a base case the nominal 20-MW/m2 neutron first-wall loading design, CRFPR(20), the cost and technology impact of lower- wall-loading designs are also examined. The additional detail developed as part of this follow-on study also allows the cost estimates to be refined. The cost impact of multiplexing lower-wall-loading FPCs into a ~ lOOO-MWe(net) plant is also examined. The CRFPR(20) design remains based on a PbLi-cooled FPC with pressurized-water used as a coolant for first-wall, pumped-limiter, and structural-shield systems. Single-piece FPC maintenance of this steady-state power plant is envisaged and evaluated on the basis of a preliminary layout of the reactor building. This follow-on study also develops the groundwork for assessing the feasibility and impact of impurity/ash control by magnetic divertors as an alternative to previously considered pumpefl- limiter systems. Lastly, directions for future, moreV detailed power-plant designs based on the Reversed-Field Pinch are suggested. 1. INTRODUCTION 1.1. Background Recent studies1'2 of the principal fusion concepts as electric power plants indicate costs of electricity [COE(mills/kWeh)] that are at least 1.5-2.0 times greater than alternative nuclear energy sources. Since a majority of the total direct cost for these designs is projected to lie in the Reactor Plant Equipment cost account (i.e., Account 22., 56% of total direct cost for STARFIRE1 and 64% for MARS2), compared to 25-30% for a light-water fission reactor,3 the most significant and direct reductions In cost can be made by reducing the size (mass) and complexity of the fusion power core (FPC, i.e., plasma chamber, first wall, blanket, shield, magnets, and structure) and related support equipment. Typically, the FPC "mass utilization" (e.g., FPC mass divided by gross thermal power) for STARFIRE and MARS is, respectively, 5.7 and 6.8 tonne/MWt, compared to ~ 0.3-0.4 for Pressurized-Water Fission Reactors (PWRs), and reductions in this figure of merit by at least a factor of 2-3 for a 1000-MWe(net)-class device are deemed necessary for competitive fusion power.H » A recent investigation6 into the role of FPC power density, mass utilization or "mass power density," has concluded that for the latter parameter a value above ~ 100 kWe(net)/tonne would give fusion a competitive position with respect to PWRs, where the latter ratio of net electric power to FPC mass has recently been suggested6 as a measure of FPC performance. Both "nui^s utilization" and "mass power density" are used in this report, with a preference for the former because of a decoupling from quantities such as thermal- conversion efficiency and recirculating power fraction, which are not entirely related to the FPC. Within limits, neverthelesst both quantities are useful indicators of device performance.6 The reduction in development cost and the enhanced probability for success because of a more flexible, affordable development path have also been suggested6 as reasons for pursuing low-mass-utilization or high-mass-power- density systems, particularly if such systems can be developed at low unit powers. Although these benefits are not easily quantified at present, the capability to extend learning curves for both physics and technology and to build rapidly and less expensively an operational database with which to assess the critical issue of plant availability also are strong reasons for emphasizing low-unit-power, high-mass-power-density approaches. A number of preconceptual and framework studies of high-mass-power-density fusion systems have been reported.7"10 Because of an ability to confine stable, high-beta plasma by self-generated poloidal fields, the Reversed-Field Pinch (RFP) offers a route to improved FPC mass power density, mass utilization, and cost based on an encouraging but developing physics database.10*11 The Ref. 10 Compact RFP Reactor (CRFPR) designs used each of two relatively independent elements in the prescription for low-mass-utilization or high-raass-powet—density FPCs: a) increased plasma power density or fusion-neutron first-wall loading, and b) reduced blanket, shield, and coil mass (and volume) allowed by the use of resistive (copper-alloy) coils. The potential for significant shrinkage of the FPC physical size, mass, and cost by using resistive copper coils without recirculating a large fraction of the gross electric power generated to supply ohmic losses in those coils if generally characteristic rf poloidal-field- dominated confinement systems12 like the RFP. The qualities of the RFP that permit efficient plasma confinement by resistive coils positioned outside a thin tritium-breeding, heat-recovering blanket and (nominal) shield also allow high engineering beta (i.e., plasma pressure normalized to the magnetic field pressure at the confining coils). Central to maintaining this high engineering beta throughout the DT burn is the postulate of a low-frequency (~ 10s Hz) oscillating-field drive for sustaining the large plasma currents against ohmic dissipation; the close coupling of toroidal and poloidal circuits through the plasma projects a unique current- drive mechanism for the RFP, called "F-0 pumping" after the F = B(j)(rp)/<B(j)> and G = BQ(rp)/<Bj,> parameters commonly used to define the Taylor near-minimum- energy state.13»1'* In these expressions, the toroidal field is B., the poloidal field is BQ, x~ is the plasma minor radius, and <> denotes an average over the plasma volume. Although partial tests of F-0 pumping are encouraging,11^5 clear demonstration of this non-intrusive current drive must await improved (i.e., higher-current, hotter, less-resistive) RFPs. Lastly, the plasma current density in RFPs is sufficient to give ohmic heating to DT ignition, thereby eliminating the need for auxiliary heating. The RFP characteristics that give high-p, plasma-physics-decoupled aspect ratio, F-0 pumping current drive, ignition by ohmic heating alone, and low- field/low-current coils combine to offer a potential for improved FPC design that, if needed, can far exceed the threshold for improvement suggested in Refs. 4 aud 6. Figure 1-1 summarizes these RFP characteristics in the form of a TOKAMAK RFP QJ 0.5 CD CQ 0.2 0.4 0.6 0.8 1.0 0.2 0.4 0.6 0.8 1.0 RADIUS.r/rp RADIUS ,r/rp 2.0 LINER 0 0.2 0.4 0.6 0.8 1.0 0.2 0.4 0.6 0.8 1.0 RADIUS.r/rp RADIUS,r/rp Fig. 1-1. Comparison of magnetic-field and q = (B,h/B0)(rp/Rx) profiles in the minor radial dimension for the RFP and the tokamak. Shown also is an illustration of the natural tendency of B.
Details
-
File Typepdf
-
Upload Time-
-
Content LanguagesEnglish
-
Upload UserAnonymous/Not logged-in
-
File Pages249 Page
-
File Size-