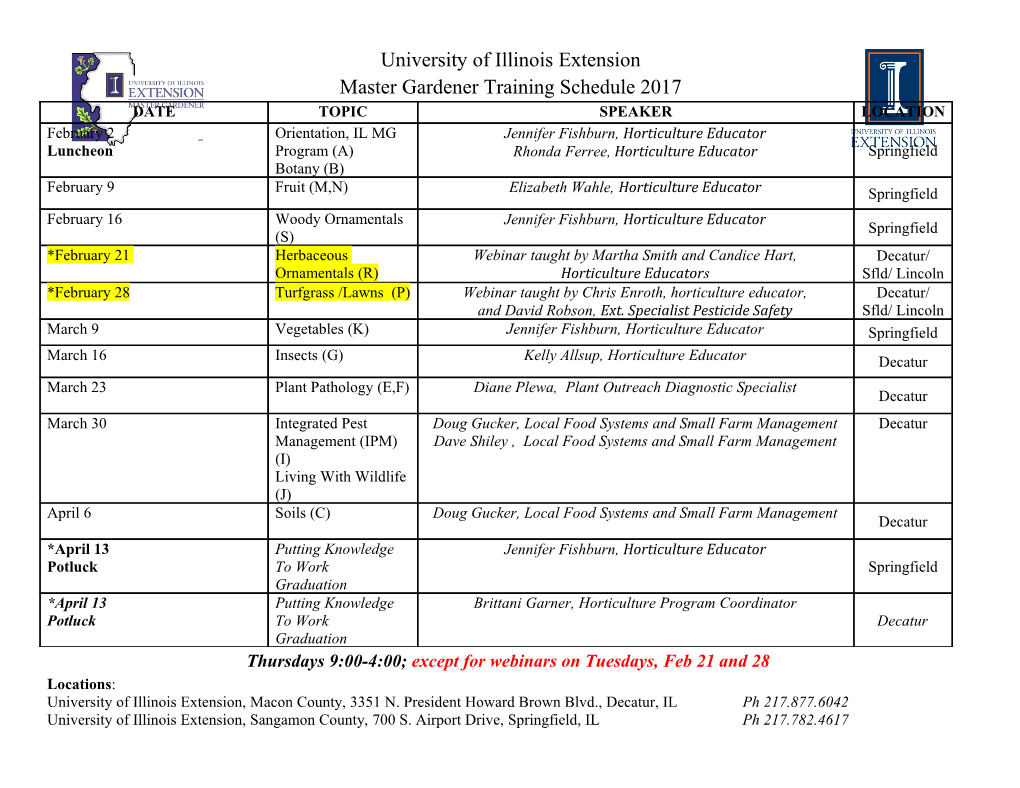
High-Energy Astrophysics and Gas-Filled Ionization Detectors Scott Griffiths High-Energy Astrophysics • High-energy astrophysical phenomena emit x-rays • X-rays are less susceptible to scattering from interstellar dust • X-rays are often emitted from ultraluminous sources, which allows observation of distant objects • X-ray data can be combined with optical, IR, and radio band data • Typical x-ray emitting astrophysical objects: – Black holes and AGNs – Neutron Stars – Supernovae and SNRs – Gamma Ray Bursts (GRBs) Grazing Incidence Optics The Center of the Milky Way • X-rays from Chandra are blue and violet • Near-IR from Hubble is in yellow • Far-IR from Spitzer is in red Supernova Remnants (SNRs) Tycho’s SNR: Type 1a G292.0+1.8: Type II Credit: NASA/CXC/Rutgers/J.Warren & J.Hughes et al. Credit: NASA/CXC/Rutgers/J.Hughes et al. Black Hole Accretion Disks Selection of X-ray Detector for BRP • Objective of BRP detector – To measure the polarization of x-rays emitted from astrophysical sources, such as black holes • Requirements for BRP detector – Low power! (1.6 W) – Moderate cost – Small size – Efficient counting • Detector of choice: Multiwire Proportional Counter (MWPC) Overview of Ionization Detectors All ionization detectors operate by incident radiation ionizing a fill-gas: 1. Radiation enters detector through window 2. Radiation ionizes several (possibly tens) of fill gas atoms and creates electron and positive ion pairs 3. The lightweight electrons drift towards the positively charged anode, while positively charged ions drift towards the negative cathode Types of Ionization Detectors • Classic designs – Ionization Chamber – Proportional Counter (PC) – Geiger-Müller Counter • Modern Designs – Multiwire Proportional Counter (MWPC) – Drift Chamber – Time Projection Chamber (TPC) – Liquid Ionization Detector (LID) – Micropattern Gas Detectors • Microstrip Gas Chamber (MSGC), Gas Electron Multiplier (GEM), Resistive Plate Chamber (RPC) Differences Between the Classic Gas-Filled Detectors • Operating voltage • Amplification • Spectral content • Dead time • Ionization Chamber: – No Amplification • Geiger Counter: – No spectral info – Long dead time (100 µs) Townsend Avalanche & Pulse Formation Note that the Townsend avalanche occurs almost entirely within a few wire radii of the anode wire Townsend Avalanche & Pulse Formation A signal is created by: 1. Absorption of the electron cascade by the anode 2. Induced charge in the anode and cathode from the motion of the electrons and ions • Only 1% of the induced signal is from the electrons • Readout of the ion-induced signal produces more consistent pulse amplitudes for monoenergetic radiation Classic Cylindrical Ionization Detector • The classic ionization detector has a single anode wire in a grounded cylindrical housing • Radiation enters the detector through a thin window (often mylar), which may be at the end of the cylinder • The detector may be closed, or may flow gas Fill Gas Interactions • Excitation: X + γ → X* + γ – Incident radiation causes an electron to transition to a more energetic state (resonant effect) – The excited electron emits a photon as it drops back down to its previous, lower energy state – The emitted photon may cause secondary ionization • Ionization: X + γ → X+ + e– + γ – Incident radiation ionizes a fill-gas atom (non-resonant effect) • Penning Effect • Molecular Ion Formation Fill Gas Selection Considerations • Gas amplification! • Energy Resolution – Determined by W (eV/ion), Fano factor F (empirical constant expressing fluctuation in the # of ion pairs created), and multiplication variance b 푊(퐹+푏) 푅푒푠표푙푢푡푖표푛 (퐹푊퐻푀) = 2.35 퐸 – – • Electron Affinity: X + e → X + γ – The fill gas must not contain large quantities of electronegative gases (O2, CO2, H2O, etc.) • Diffusion and Drift Characteristics – Low diffusion means good spatial resolution – Fast drift means fast collection times Fill Gas Selection Considerations Continued • Quench Gas – A small amount of (typically) organic quench gas can be used to preferentially absorb photons emitted from excitation and dissipate this energy in non-ionizing modes – Prevents continuous discharge and allows for higher gas gains (~106 instead of ~104) • Build-up Prevention Gas – Removes build-up from spent organic quench gas, which can cause continuous discharge • Environmental Impact (No Freon!) • Cost ($$$) Multiwire Proportional Counters Typical MWPC configurations. Note that signals may be read from the anode array, cathode arrays, or both. Wires may be ganged together to reduce readout electronics complexity. Wires are typically high-uniformity gold-plated Tungsten, usually 10-50 microns in diameter. Anode wire spacing is typically 2 mm, while cathode wires are typically spaced approx. 0.5 mm apart. Field Geometry, Anodes Only Field Geometry with Cathode Arrays BRP Frontend Electronics BRP Fill Gas Selection • P10 = 90% Argon, 10% Methane • P10 selected for BRP because of its high gain, stability, and energy resolution • P10 has an average ionization energy of 26 eV, which implies a 0.5 keV x- ray can ionize at most 19 atoms of fill gas • This implies a Fano factor of approximately 0.2 MWPC Stacked PCB Design Single Wire PC Mounted on CF Flange Preamps Test Pulser Vacuum Chamber High-Voltage (2 kV) Power Manson X-ray Tube Controls Shaping Amp Ion Pump Controls Gas Flow Meter MCA Data for Multiwire PC, V 1.80 kV, SA Gain 30 Counts 400 Fe-55 Kα Line Escape Peak 300 200 100 Channel 100 200 300 400 500 Proportional Counter Gain Curves 1000000 100000 10000 Gain 1000 100 10 1400 1500 1600 1700 1800 1900 2000 Power Supply Voltage (V) 25 μm SWPC - SHV Posts 50 μm SWPC - PCB 25 μm SWPC - PCB MWPC - Cathode 0V MWPC - Cathode 200V Primary References: .
Details
-
File Typepdf
-
Upload Time-
-
Content LanguagesEnglish
-
Upload UserAnonymous/Not logged-in
-
File Pages26 Page
-
File Size-