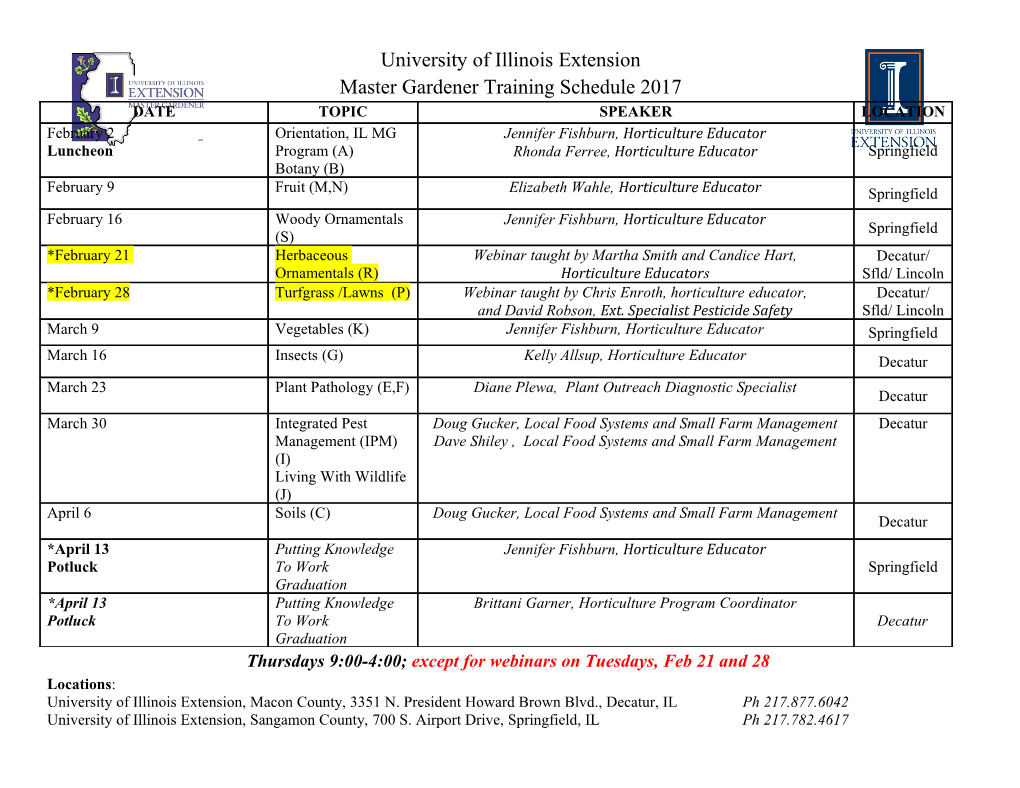
895 A publication of CHEMICAL ENGINEERING TRANSACTIONS VOL. 61, 2017 The Italian Association of Chemical Engineering Online at www.aidic.it/cet Guest Editors: Petar S Varbanov, Rongxin Su, Hon Loong Lam, Xia Liu, Jiří J Klemeš Copyright © 2017, AIDIC Servizi S.r.l. ISBN 978-88-95608-51-8; ISSN 2283-9216 DOI: 10.3303/CET1761147 Hydrogenation of Biomass-Derived Levulinic Acid to Gamma- Valerolactone Using Polymer-Based Metal-Containing Catalysts Linda Zh. Nikoshvili*, Igor I. Protsenko, Dialia A. Abusuek, Anna O. Zaykovskaya, Alexey V. Bykov, Valentina G. Matveeva, Esther M. Sulman Tver Technical University, A.Nikitina str. 22, 170026, Tver, Russian Federation [email protected] Nowadays the development of effective catalytic systems of selective hydrogenation of levulinic acid (LA) to gamma -valerolactone (GVL) is of high importance. However, there is a lack of data concerning the use of polymer -based catalyst in this process. The possibility to use of Ru-containing catalysts on the basis of hypercrosslinked polystyrene (HPS) in hydrogenation of LA to GVL is discussed. Catalyst 5%-Ru/HPS is shown to be highly active and selective in hydrogenation of LA in aqueous medium (yield of GVL more than 99%) at mild reaction conditions (90 °C, 2 MPa of hydrogen partial pressure) and the absence of co-catalysts. 1. Introduction Global petro-chemical market faces increasing competition and a dependency on external sources, and is under considerable cost and ecological pressure. Biomass has received considerable attention as a sustainable feedstock that can replace diminishing fossil fuels for the production of energy, especially for the transportation sector. The first-generation biofuels are produced from sugars, starches and vegetable oils; however, the limited availability of starting materials (due to the restricted amounts of fertile soils) and competition with food from the feedstocks are limiting factors for its production (Alonso et al., 2010). The advanced biofuels of second generation are mainly produced from lignocellulosic biomass (Figure 1), one of the most inexpensive and abundant raw materials (Climent et al., 2014). 40-50 % of the lignocellulose consists of cellulose, the most valuable component since it can be converted into various platform molecules, including levulinic acid (LA) (Weingarten et al., 2012), which can be obtained from cellulosic biomass via acid hydrolysis (Sivasubramaniam and Amin, 2015). Hydrogenation of LA to gamma-valerolactone (GVL) is one of the most promising reactions in the field of biomass valorization to fine chemicals and liquid transportation fuels (Alonso et al., 2013), and GVL is one of the most widespread lactones, which can be obtained by hydrogenation of LA (De Souza et al., 2014). Figure 1: General scheme of biomass processing to GVL – a platform-chemical for liquid biofuels Please cite this article as: Nikoshvili L.Z., Protsenko I.I., Abusuek D.A., Zaykovskaya A.O., Bykov A.V., Matveeva V., Sulman E., 2017, Hydrogenation of biomass-derived levulinic acid to gamma-valerolactone using polymer-based metal-containing catalysts, Chemical Engineering Transactions, 61, 895-900 DOI:10.3303/CET1761147 896 Due to high interest towards GVL, its efficient production is currently a topic of intensive research (Piskun et al., 2016). Investigations are mainly focused on hydrogenation of LA (Al-Shaal et al., 2016) and its esters (Cai et al., 2016) by molecular hydrogen in the presence of metal catalysts. Application of supported metal catalysts is especially advantageous owing to simplicity of product recovery and catalyst recycling (Zhang et al., 2015). LA can not only serve as a precursor in the synthesis of GVL (Carvalheiro et al., 2008), it can be also transformed to 2-methyltetrahydrofuran, which is a fuel additive. It is noteworthy that 2- methyltetrahydrofuran is permissible to mix up to 70 % with gasoline without causing harm to the internal combustion engines, and similar mileage is reached. Although there is a possibility of direct LA transformation to 2-methyltetrahydrofuran, improved yields can be achieved by indirect pathways, which proceed through GVL as an intermediate (Huber and Corma, 2007). One of the key issues of production of new types of fuels is the proper choice of reagents and reaction conditions. 5 %-Ru/C is one of the most widespread heterogeneous catalysts of LA hydrogenation (Selva et al., 2013). Different solvents (methanol, ethanol, butanol, 1,4-dioxane and water) can be used to achieve high conversion of LA (Al-Shaal et al., 2012). Besides the 5 %-Ru/C, which was shown to provide high yields of GVL at relatively mild reaction conditions (Protsenko et al., 2016), a number of the heterogeneous catalysts intended for the synthesis of GVL from LA has been developed as a result of numerous studies, e.g. the catalysts on the basis of noble metals such as Pd (Wright and Palkovits, 2012) and Pt (Upare et al., 2011) deposited on inorganic supports. However, it is known that the main problem of industrial biofuel production is high cost, which can be due to the necessity of the use of catalysts containing platinum, palladium and ruthenium. As regards to this issue, monometallic catalysts on the basis of Ni (Fu et al., 2016), Co (Zhang et al., 2013), Cu (Putrakumar et al., 2015), Fe (Long et al., 2015) and bimetallic ones (Ni-Cu (Obregón et al., 2014), Cu-Cr (Yan and Chen, 2013), Cu-Fe (Yan and Chen, 2014)) are perspective. It is noteworthy that up to date there is no data on the use of polymer-based catalysts in hydrogenation of LA to GVL. Thus the methodology of this work is based on the use of hypercrosslinked polystyrene (HPS) as a support for the synthesis of heterogeneous catalysts of LA hydrogenation owing to the ability of HPS to stabilization of metal-containing nanoparticles. Earlier it was shown that Ru/HPS, especially the catalysts based on HPS of MN100 type containing amino-groups, can serve as an alternative to the commercial 5 %-Ru/C catalyst (Protsenko et al., 2016), however the bounds of high GVL yield for Ru/HPS catalysts were not studied. In the framework of this study we investigated the influence of reaction conditions on the behavior of the most promising HPS-based catalyst, i.e. 5 %-Ru/MN100. Besides, the series of catalysts containing 5 wt.% of non-noble metals (Co, Fe, Ni, Cu and Zn) was synthesized using MN100 as a support and corresponding metal acetates as precursors. However, these catalysts revealed marginal conversion of LA in aqueous medium at 200 °C and 5 MPa of hydrogen partial pressure at the absence of any co-catalysts, as it was also shown elsewhere for Cu/C (Xu et al., 2016). Thus, this work is focused on physicochemical characterisation and detailed investigation of catalytic properties of 5 %-Ru/MN100. 2. Experimental 2.1 Materials HPS was purchased from Purolite Int. (U.K.), as Macronet MN100. Ruthenium hydroxychloride (Ru(OH)Cl3) was purchased from Aurat Ltd. (Moscow, Russian Federation). LA (≥ 98 %) was purchased from Merck KGaA, Germany. GVL (ReagentPlus®, 99 %) was purchased from Sigma-Aldrich. Reagent-grade THF, methanol, acetone and hydrogen peroxide were purchased from Sigma-Aldrich and were used as received. Sodium hydroxide was obtained from Reakhim (Moscow, Russian Federation). Reagent grade hydrogen of 99.999 % purity was received from AGA. Distilled water was purified with an Elsi-Aqua (Elsico, Moscow, Russia) water purification system. 2.2 Catalyst synthesis HPS-based Ru-containing catalyst was synthesized via conventional wet-impregnation method according to the procedure described elsewhere (Sapunov et al., 2013). In a typical experiment, 3 g of pre-treated (washed with distilled water and then with acetone to remove chloride and iron ions), dried and crushed (< 63 μm) granules of HPS were impregnated with 7 mL of the solvent mixture consisting of THF (5 mL), methanol (1 mL) and water (1 mL), with dissolved therein calculated amount of ruthenium hydroxychloride (Ru(OH)Cl3) for 10 min. The Ru- containing polymer was dried at 70 °C for 1 h, refluxed in 21 mL of NaOH aqueous solution (concentration 0.1 mol/L), then 2 mL of hydrogen peroxide was added at continuous stirring. The catalyst was washed with distilled water until pH 6.4 – 7.0, and lack of response to chloride ions, and dried again at 70 °C. In this way, 5 %- Ru/MN100 was synthesized. The catalyst was reduced in hydrogen flow at a temperature of 300 °C and H2 flow rate of 100 mL/min during 2 h (designated as 5 %-Ru/MN100-R). 897 2.3 Procedure of catalytic testing The hydrogenation reaction (Figure 2) was carried out in Parr Series 5000 Multiple Reactor System at a stirring rate of 1500 rpm, at variation of such process parameters as temperature (70 – 120 °C) and hydrogen partial pressure (1 – 5 MPa). In each experiment, LA loading was 1 g and the catalyst loading was 0.01 g. Water was used as a solvent (volume of liquid phase was 50 mL). Samples of reaction mixture were analyzed via HPLC method using absolute calibration method. Figure 2: Scheme of catalytic hydrogenation of LA to GVL and subsequent products 2.4 Methods Synthesized 5 %-Ru/MN100 was characterized by liquid nitrogen physisorption, X-ray photoelectron spectroscopy (XPS), transmission electron microscopy (TEM) and scanning electron microscopy (SEM). Liquid nitrogen physisorption was carried out using Beckman Coulter SA 3100 (Coulter Corporation, USA). Prior to the analysis, samples were degassed in Becman Coulter SA-PREP at 120C in vacuum for 1 h. Weight of each sample was above 0.1 g. XPS data were obtained using Mg Kα (h = 1253.6 eV) radiation with ES-2403 spectrometer (Institute for Analytic Instrumentation of RAS, St. Petersburg, Russia) equipped with energy analyzer PHOIBOS 100-MCD5 (SPECS, Germany) and X-Ray source XR-50 (SPECS, Germany).
Details
-
File Typepdf
-
Upload Time-
-
Content LanguagesEnglish
-
Upload UserAnonymous/Not logged-in
-
File Pages6 Page
-
File Size-