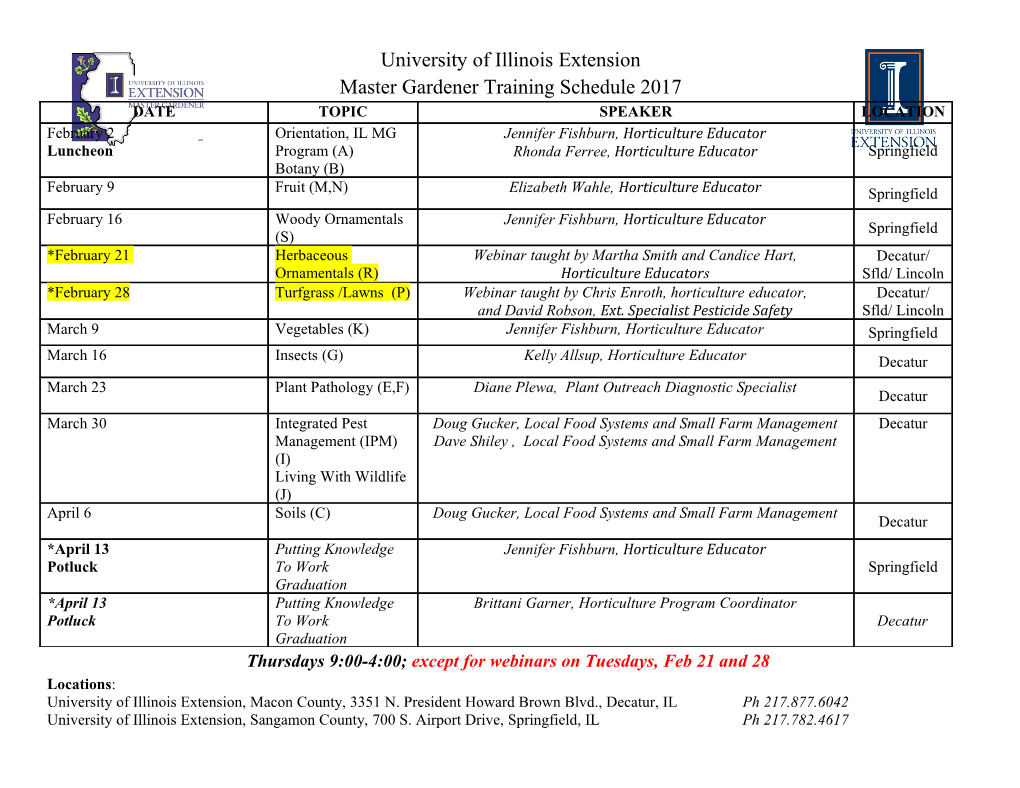
Photon upconversion for thin film solar cells ISBN 978-90-393-5828-3 Photon upconversion for thin film solar cells Foton upconversie voor dunne film zonnecellen (met samenvatting in het Nederlands) Proefschrift ter verkrijging van de graad doctor aan de Universiteit Utrecht op gezag van rector magnificus, prof.dr. G.J. van der Zwaan, ingevolge het besluit van het college voor promoties in het openbaar de verdedigen op maandag 17 september 2012 des middags te 2.30 uur door Jessica de Wild geboren op 15 september 1982 te Spijkenisse Promotoren Prof.dr. R.E.I. Schropp Prof.dr. A. Meijerink Co-promotoren Dr. J.K. Rath Dr. W.G.J.H.M. van Sark The work described in this thesis was financially supported by NL Agency (Agentschap NL) of the Ministry of Economic Affairs, Agriculture and Innovation of The Netherlands: program EOS: LT NEO (New Energy Research). Contents 1 Introduction and theory 9 1.1 Energy losses in solar cells . .9 1.2 Spectral conversion . 10 1.3 Available upconverter materials . 13 1.3.1 Organic Molecules . 13 1.3.2 Lanthanides . 15 1.4 Upconverter efficiency . 18 1.5 Application of upconverters to solar cells . 19 1.6 Light intensity and efficient upconversion . 20 1.7 Summary and outline . 22 2 Experimental methods 25 Abstract . 25 2.1 Deposition techniques . 26 2.1.1 PECVD . 26 2.1.2 Magnetron Sputtering . 26 2.1.3 Evaporation . 26 2.2 Characterization techniques . 27 2.3 Material characterization . 27 2.3.1 Reflection transmission . 27 2.3.2 Hall Measurements . 27 2.3.3 4-point probe . 28 2.3.4 Integrating sphere . 28 2.3.5 X-ray diffraction . 29 2.3.6 Spectroscopy . 30 2.4 Solar Cell Characterization . 32 2.4.1 Solar Simulator . 32 2.4.2 Spectral Response . 33 2.4.3 Fourier Transform Photo spectroscopy . 34 6 Contents 3 Upconverter material: NaYF4 35 Abstract . 35 3.1 Introduction . 36 3.2 Experimental . 38 3.3 Results and discussion . 39 3.3.1 Absorption spectra . 39 3.3.2 Luminescence spectra . 40 3.3.3 Power Dependence Upconversion . 41 3.3.4 Energy Transfer . 42 3.3.5 Temperature dependence . 46 3.4 Conclusions . 48 4 Investigation of upconversion efficiencies for low power excitation 49 Abstract . 49 4.1 Introduction . 50 4.2 Experimental . 51 4.3 Upconversion in Gd2O2S ........................ 52 4.3.1 Absorption spectra . 53 4.3.2 Luminescence spectra . 54 4.3.3 Power dependence upconversion . 56 4.3.4 Comparison with β-NaYF4 .................. 59 3+ 3+ 4.4 Upconversion in β-NaYF4 : Er , Yb nano-crystals near gold nanorods . 61 4.4.1 Gold nanorods synthesis . 62 3+ 3+ 4.4.2 NaYF4 :Er , Yb NCs and gold nanorod aggregates . 64 4.4.3 Luminescence . 64 4.4.4 Lifetime . 66 4.5 Discussion and conclusion . 67 5 Thin film a-Si:H Solar Cells 69 Abstract . 69 5.1 Introduction . 70 5.2 Experimental . 70 5.3 Results and discussion . 71 5.3.1 TCO . 71 5.3.2 Solar Cells . 74 5.4 Conclusion . 81 6 Solar Cells with Upconverter 83 Abstract . 83 6.1 Introduction . 84 6.2 Experimental . 85 6.3 Results and discussion . 86 6.3.1 Solar cells with NaYF4 upconverter . 86 Contents 7 6.3.2 Device modifications . 89 6.3.3 Laser light and equivalent number of suns . 92 6.3.4 Broad band excitation . 92 6.3.5 Upconversion luminescence efficiency . 97 6.4 Conclusions . 98 Bibliography 99 Summary 111 Nederlandse samenvatting 115 List of publications 121 Dankwoord 123 Curriculum Vitae 125 8 Contents Chapter 1 Introduction and theory 1.1 Energy losses in solar cells The ever growing energy demand combined with the decreasing resources of fossil fuels makes it evident that there is a need for a transition towards renewable energy supply technologies. Solar cells are projected to make a significant contribution to the future of renewable energy. Solar energy can provide sufficient energy to fill the gap left by fossil fuels using only a limited fraction of the earth surface area [1]. The relatively high efficiency and flexibility allowing for small scale application in rural areas and on roof tops as well as for large grid connected solar energy farms contributes to the rapidly growing solar energy market. To sustain the present market growth, the cost of solar electricity has to decrease further. Cost reduction can be achieved by combining low-cost production technologies, cheaper materials, and increased efficiency of the solar cells. The maximum efficiency of solar cells is largely determined by the band gap of the semiconductor material. Single junc- tion solar cells suffer from intrinsic efficiency losses, see figure 1.1. Among other losses, the inability to absorb photons with an energy lower than the band gap (1) and relaxation losses of excess energy of absorbed high energy photons (2) make up at least ~65% of the total energy loss in single junction solar cells [2]. These spectral mismatch losses are called transmission and thermalization losses. Since the sun is a polychromatic light source there is a trade off between these losses, resulting in a fundamentally determined maximum efficiency as a function of band gap energy. This maximum energy efficiency of single junction solar cells was al- ready determined by Shockley and Queisser in the 1960’s and is now referred to as Shockley-Queisser (SQ) limit. The highest efficiencies reported for single junction solar cells such as c-Si and GaAs are presently approaching the maximum effi- ciency set by the SQ limit, which is 33% [3]. Further improvement of these high efficiencies becomes increasingly complicated. Expensive techniques are required for incremental efficiency improvements. Clearly, fundamentally different concepts are necessary to increase the efficiency beyond the SQ limit. Different options are 10 Introduction and theory being explored, which can be divided in concepts based on adapting the solar cell and concepts aimed at adapting the solar spectrum. In both cases multiple energy levels are utilized to reduce transmission and thermalization losses [4, 5]. The most common and proven method to overcome the SQ limit is realized in multi-junction solar cells, consisting of a stack of solar cells with different band gaps. Each type of solar cell converts a part of the solar spectrum with high efficiencies, resulting in record energy efficiencies above 40%, theoretically even higher [3]. Because these cells are normally electrically connected in series, the solar cell with the lowest current is limiting the efficiency. This makes these types of solar cells sensitive to spectral changes, which are due to seasonal changes, daily changes, and sky con- ditions (clear/cloudy) [6]. Another device structure which is investigated to reach higher efficiency by reducing the transmission losses is the intermediate band so- lar cell [7, 8]. With an intermediate band, sub band gap photons are absorbed to and from the intermediate band. The photons are absorbed parallel to the operating cell, decreasing the spectral selectivity and increasing the photogener- ated current. Device structures reducing the thermalization losses are based on hot carrier extraction and multiple carrier generation. Hot carrier extraction aims at collection of the photogenerated carriers before they thermalize. Hereby the voltage of the cell is increased [9]. With multiple exciton generation, high energy photons undergo impact ionization and multiple carriers are generated, increasing the photogenerated current is increased. Multiple carrier generation mainly occurs in quantum dots [10, 11]. The latter concepts are still under development at the materials level and the efficiency for some of the effects is controversial [12, 13]. 1.2 Spectral conversion An alternative approach is spectral conversion aimed at modification of the solar spectrum to achieve a better match with the wavelength dependent conversion efficiency of the solar cell. The advantage of spectral conversion is that this can be applied to existing solar cells and that optimization of the solar cell and spec- tral converter can be done separately. Different types of spectral conversion can be distinguished: two low energy (sub band gap) photons are combined to one high energy photon (upconversion) or one high energy photon is transformed into one (downshifting) or two (downconversion or quantum cutting) lower energy pho- tons, see figure 1.2. Downshifting can give a marginal efficiency increase by shifting photons to a spectral region where the solar cell has a higher efficiency. Up- and downconversion however can raise the efficiency above the SQ limit. Research on spectral conversion is focused on organic dyes and quantum dots for down- shifting and lanthanide ions and transition metal ion for up- and downconversion [15, 16, 17]. Especially upconversion attracts a lot of attention recently, because it involves low energy photons that are not absorbed by the solar cells: the trans- mitted photons. An upconversion layer can be placed at the back of the solar cells and by converting part of the transmitted photons to wavelengths that can Spectral conversion 11 2 5 hν = Eg Eg 1 hν > Eg E hν < Eg 3 C 3 E2 Eg 4 E 5 IL E1 E EV 2 Band diagram (a) Upconverter (b) Figure 1.1: Losses in single junction solar cells (a). The thermalization and transmission losses are represented by 1 and 2. Other losses are contact losses (3), recombination losses (4) and junction losses (5). With an upconverter the transmission losses can be reduced by adding an intermediate energy band (EIL) in the band gap. After subsequent absorption of two low energy photons, a higher energy photon is emitted (b). The upconverter is electrically isolated. Figure (a) adapted from ref [14]. be absorbed, it is relatively straightforward to demonstrate a positive contribu- tion from the upconversion layer, even if the upconversion efficiency is low.
Details
-
File Typepdf
-
Upload Time-
-
Content LanguagesEnglish
-
Upload UserAnonymous/Not logged-in
-
File Pages127 Page
-
File Size-