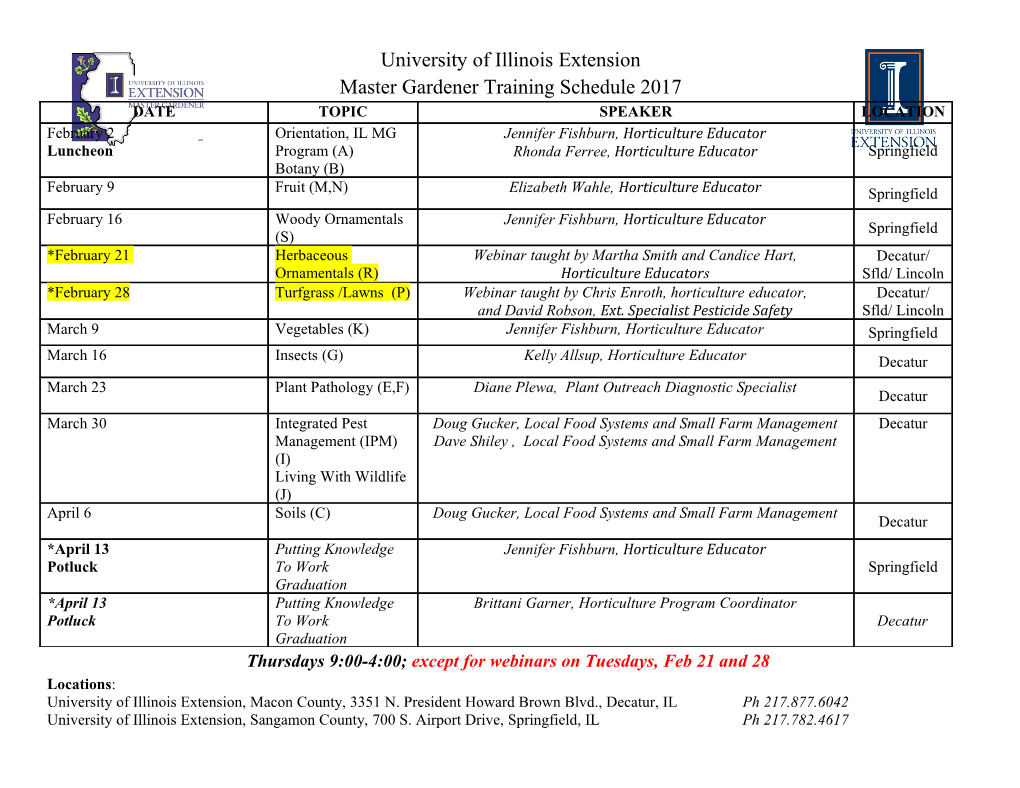
CALCIUM IMAGING OF DEVELOPING PROPRIOCEPTIVE DORSAL ROOT GANGLION NEURONS A thesis submitted in partial fulfillment of the requirements for the degree of Master of Science By KAITLYN LOUISE PARKES B.A., University of Alabama at Birmingham, 2014 2019 Wright State University WRIGHT STATE UNIVERSITY GRADUATE SCHOOL April 24, 2019 I HEREBY RECOMMEND THAT THE THESIS PREPARED UNDER MY SUPERVISION BY Kaitlyn Louise Parkes ENTITLED Calcium Imaging of Developing Proprioceptive Dorsal Root Ganglion Neurons BE ACCEPTED IN PARTIAL FULFILLMENT OF THE REQUIREMENTS FOR THE DEGREE OF Master of Science. David R. Ladle, Ph.D. Thesis Director Eric S. Bennett, Ph.D Department Chair Department of Neuroscience, Cell Biology and Physiology Committee on Final Examination David R. Ladle, Ph.D. Patrick M. Sonner, Ph.D. Gary L. Nieder, Ph.D. Barry Milligan, Ph.D. Interim Dean of the Graduate School ABSTRACT Parkes, Kaitlyn Louise. M.S. Department of Neuroscience, Cell Biology and Physiology, Wright State University, 2019. Calcium Imaging in Developing Proprioceptive Dorsal Root Ganglion Neurons. Proprioception is an important sensation capable due to proprioceptive sensory neuron afferents found in muscles in the periphery being processed by the central nervous system. The nervous system is a complex system that continues to develop and mature as an animal ages. Much is not known of proprioceptive neurons and how they develop with time. Calcium is an important molecule in maintaining action potentials and homeostasis in neurons which can be studied to understand a variety of things about a neuron. This study uses genetically encoded calcium indicators to tag parvalbumin positive cells in the dorsal root ganglion (DRG) in order to image the calcium handling in these cells. Five parameters were measured across three time points to provide quantifiable results as to how calcium handling changes as mice age. We found that of the five parameters investigated, peak amplitude was the only significant difference between P5/6 and adult mice. There was a downward trend in the peak amplitude as the mice aged. iii TABLE OF CONTENTS Page I. Introduction……………………………………………………….………..1 Proprioceptive Neuron Subtypes……………………..……….…...1 Cellular and Behavioral Maturation During Postnatal Development……………………………………………………….5 Calcium Regulation in Neurons…………..………………………..6 II. Materials and Methods……………………………………………….…….8 Animals………………………………………………………….…8 Ex Vivo Quadriceps Nerve and L2-L4 DRG Preparation…………9 Calcium Imaging………………………………………………….11 Data processing…………………………………………………...12 Statistics…………………………………………………………..13 III. Results……………………………………………………………….…....14 Calcium Imaging Data Acquisition……………………………....14 Description of Proprioceptive Calcium Transients………….…...14 IV. Discussion……………………………………………………….….…….22 V. References ………………………………………………………….….…25 v LIST OF FIGURES Figure Page 1. Calcium imaging of proprioceptive DRG neurons………………….……..17 2. Box and whisker plots of the five parameters studied………………….….20 3. Representative calcium transients for each age group evaluated…….…....21 vi LIST OF TABLES Table Page 1. Gender count for each age group used for calcium imaging………………….16 2. Descriptive statistics for calcium transient variables for each studied age group……………………………………………………………………...19 vii I. Introduction Proprioceptive neurons are special sensory afferents that provide feedback to the central nervous system (CNS) on the stretch and contraction of muscles. The central nervous system integrates this feedback which allows us to sense our body’s position in space. Together with the CNS, proprioceptive neurons are responsible for the coordination of movement and the ability to complete coordinated tasks without visual aid. A study showing the difficulty of slicing a piece of bread after upper limb nerve injury is a perfect demonstration of proprioceptive input needed for daily life activities. In this study, patients with neuropathy in large-fiber sensory nerves were compared to controls in a repeat back and forward cutting motion with blocked vision. It was shown that those with neuropathy had non-linear, jagged movement demonstrating the lack of coordination of the shoulder and elbow that proprioception normally aids with (Sainburg, Ghilardi, Poizner, & Ghez, 1995). Proprioceptive Neuron Subtypes There are two classes of proprioceptive sensory neurons (PSNs) known as muscle spindle and Golgi tendon organ afferents. The cell bodies of both of these PSNs are located in the dorsal root ganglia (DRG) along with other somatosensory neurons. These PSNs differ in terms of function, peripheral location, morphology, and patterns of synapses in the spinal cord. Very early in the studies of these afferent fibers, it was apparent that, based on function and form, muscle spindles are subdivided into two categories (Hunt & Kuffler, 1951). These two subcategories were defined as type Ia and 1 II fibers. Both of these afferent fibers sense the stretch on muscle fibers, but they have unique functions that subcategorize them. On the other hand, Golgi tendon organs are innervated by type Ib afferent fibers that are located at the musculotendon junction and provide feedback on muscle tension. When looking at the function of the three special sensory afferents that comprise the proprioceptive sensory neurons, differences are apparent. Muscle spindles are stretch receptors which provide feedback on the rate and degree of elongation of muscle fibers (Zelená, 1994). Golgi tendon organs are mechanoreceptors which are sensitive to contraction and responsible for feedback regarding muscle tension (Jami, 1992; Zelená, 1994). Further functional differences between Ia and II muscle spindle afferents have also been identified. Ia fibers are activated by brief stretches and small amplitude vibration (Baldissera, Hultborn, & Illert, 1981). They are statically and dynamically responsive to both length changes in muscle fibers and the velocity of the length changes (De-Doncker, Picquet, Petit, & Falempin, 2003). Type II afferents are also responsive to static stimulation and muscle stretch, but do not respond to muscle vibration (Baldissera et al., 1981; Brown, 1981). The pattern of connections with neurons in the spinal cord also differs between muscle spindle and Golgi tendon organ neurons. When looking at the spinal cord, neuron cell bodies are found in the grey matter. The grey matter is divided into ten different domains, called laminae, based on the size and type of neurons located there (Rexed, 1952). Terminal fibers from both muscle spindles and Golgi tendon organs project into the same laminae of V, VI, and VII, but each specific PSN class (Ia, II, Ib) have nuanced differences in their connections. Ia fibers also project into laminae VIII, and IX. The most 2 dense population are in in laminae V and VI (Jankowska, 1992; Vincent et al., 2017). Entering at the dorsomedial portion of the dorsal horn, they travel directly to laminae V and VI where they further branch. Synapses in lamina VII are with inhibitory interneurons, but many fibers travel to synapse in lamina IX at the motor nuclei. Here they synapse with homonymous muscle motoneurons (those that innervate the same muscle) as well as heteronymous muscle motoneurons (innervating muscles that share a similar action of a muscle) (Brown, 1981). Group II afferents have connections that resemble that of the Ia afferents, but compared to other PNS fibers their branching once in the spinal cord is more variable. One main difference is that their presence in lamina IX is sparse (Brown, 1981; Jankowska, 1992; Vincent et al., 2017). Ib afferents remain contained in laminae V, VI, and VII with the majority being located in the medial part of laminae VI (Jankowska, 1992; Vincent et al., 2017). The afferent fiber typically enters at the dorsomedial portion of the dorsal horn with a direct path to laminae V where it then branches widely between laminas V, VI, and VII (Brown, 1981). Peripheral projections of muscle spindles (Ia and II) and Golgi tendon organs differ not only in location but in morphology. Golgi tendon organs are found in the muscular connection of a tendon or aponeurosis. GTOs are encapsulated bundles of large afferent fibers that have a spindle shape with tapered ends. These can often be bifid, with two heads at both ends, or trifid, with three heads. Their size and abundance in a muscle depends on function and size of the muscle it is innervating. For example, small muscles such as the masseter have far less GTOs than a large muscle like semitendinosus. Muscles of mastication also seem to have a lower number of GTOs than limb muscles (Jami, 1992). Muscle spindles are found in parallel with extrafusal muscle fibers, the 3 normal muscle fibers that make up the bulk of a muscle (McCloskey, 1978). They are comprised of three different intrafusal fibers (specialized muscle fibers) known as bag1, bag2, and chain fibers, encapsulated in a spiral shape. These muscle spindles are supplied by one Ia fiber and often times supplied by one or more type II fiber, but there are some spindles that receive no type II connection (Zelená, 1994). While proprioceptive neurons differ in function, location and morphology, identifying a molecular marker that is unique to any one PSN has yet to happen (Poliak, Norovich, Yamagata, Sanes, & Jessell, 2016). There are, however, known molecular markers for proprioceptors as a whole. One key distinguishing factor of the DRG cell population is the dependency on neurotropin-3 (NT-3). The proprioceptive neurons
Details
-
File Typepdf
-
Upload Time-
-
Content LanguagesEnglish
-
Upload UserAnonymous/Not logged-in
-
File Pages35 Page
-
File Size-