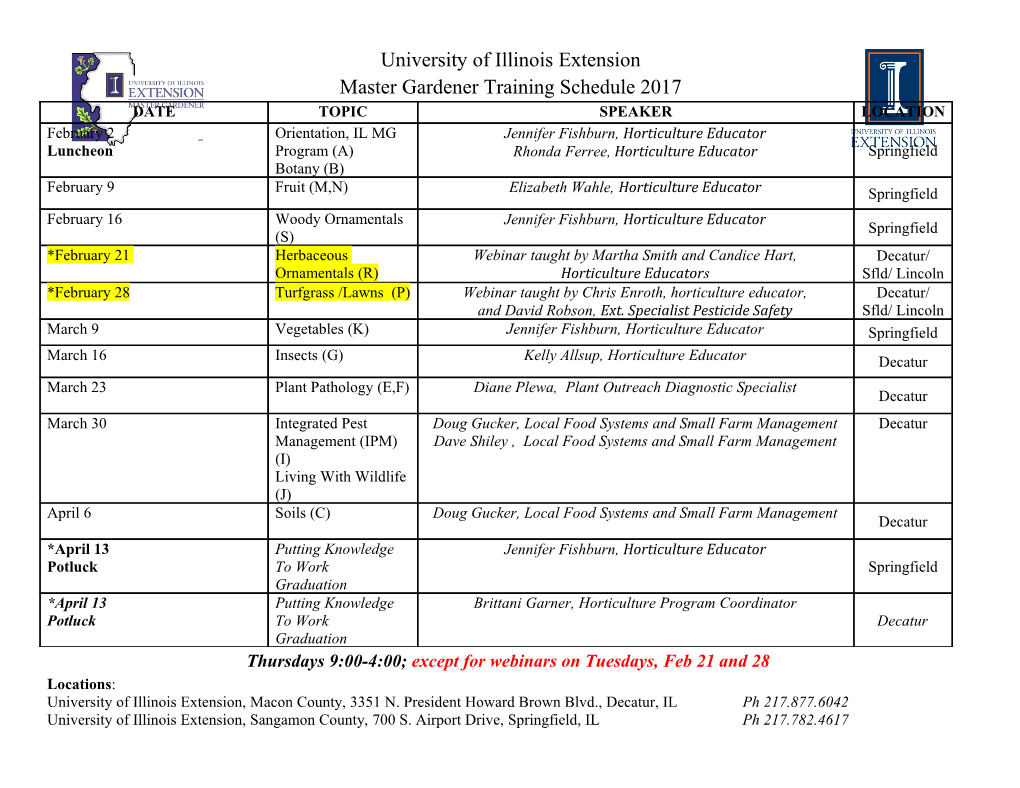
Metrics to find life from remote sensing data & their limitaons David Catling Dept. of Earth and Space Sciences/ Astrobiology Program University of Washington, Seale +NASA Earth & Space Science Fellowship student, Josh Krissansen-Toon Outline Part 1: Background: ideas of looking for life remotely Part 2: A) Looking at thermodynamic disequilibrium (in Gibbs energy/mole air) in Solar System atmospheres as a metric for life B) the kinecs of disequilibrium Part 3: Where we’re going from here PART 1: Ideas for finding life remotely: 1. Search for Extraterrestrial Intelligence (SETI) legit approach, but not the focus of this talk/meeting 2. Biogenic surfaces microbial or multicellular pigments-discussed yesterday somewhat 3. Biogenic gases Which gases? How many gases? What levels constitute life detection? Why chemical disequilibrium? Chemical disequilibrium as a sign of life? Part 1 “Kinec instability in the context of local chemical and physical condions…” Joshua Lederberg (1965) Nature More than one: “Search for…compounds in the planet’s atmosphere that are incompable on a long-term basis” James Lovelock (1965) Nature “gaseous oxygen…and atmospheric methane in extreme thermodynamic disequilibrium...are strongly suggesve of life on Earth” Carl Sagan (1993) Science Chemical disequilibrium as a sign of life? Part 1 “Kinec instability in the context of local chemical and physical condions…” Joshua Lederberg (1965) Nature More than one: “Search for…compounds in the planet’s atmosphere that are incompable on a long-term basis” James Lovelock (1965) Nature “gaseous oxygen…and atmospheric methane in extreme thermodynamic disequilibrium...are strongly suggesve of life on Earth” Carl Sagan (1993) Science Chemical disequilibrium as a sign of life? Part 1 “Kinec instability in the context of local chemical and physical condions…” Joshua Lederberg (1965) Nature More than one: “Search for…compounds in the planet’s atmosphere that are incompable on a long-term basis” James Lovelock (1965) Nature “gaseous oxygen…and atmospheric methane in extreme thermodynamic disequilibrium...are strongly suggesve of life on Earth” Carl Sagan (1993) Science Modern incarnation: Biogenic gases in reflectance spectra <0.3 ppmv O2 If photosynthesis ceased, O2 decreases exponentially to <0.4% in 21% O2 ~10 m.y. Proposed search to Earth-like exoplanets for O2 and CH4 0.1% O2 Brightness temperature (K) wavelength (μm) Disequilibrium applies to waste biogenic gases, but it’s nuanced: 1) All planetary atmospheres are in disequilibrium - Geophysics competes with biology. How much? 2) Life feeds on disequilibrium so sometimes disequilibrium might mean “no one home” i.e., uneaten free food=> no grad students no life Part 1 Atmospheric disequilibrium as a biosignature on exoplanets? 9 e.g., back-of-envelope esmate: CH4 in fixed bulk air (net) CH4 + 2O2 ! CO2 + 2H2O 0 0 0 -1 ΔGreaction = ∑Gproducts − ∑Greactants = −817.9 kJ mol Negave: thermodynamically favored, with equilibrium constant for bB + cC = gG + hH of activity product of products a ga h ⎛ −ΔG 0 ⎞ K G H exp reaction eq, 298K = = b c = ⎜ ⎟ activity product of reactants aB aC ⎝ RT ⎠ Hence, equilibrium methane in 0.21 bar O2 and 380 ppmv CO2 is: −6 (pCO2 )(aH2O ) 380 ×10 −145 pCH4 ~ 2 = 2 143.29 = 10 bar (pO2 ) Keq (0.21) ×10 -6 But actual CH4 abundance is 1.8 x 10 bar Equilibrium of each gas with fixed bulk air: O2, N2, CO2, H2O(g) Gas Abundance Equilibrium Source Detectable in abundance Galileo NIR spectra of Earth? -11 -10 CS2 10 – 10 ~0 >80% Biology; + volcanic OCS 10-10 ~0 Biology + photochemistry -11 -10 SO2 10 – 10 ~0 Volcanic + photochemistry -6 -145 CH4 1.8 × 10 10 >90% biology; + geothermal -10 -9 -60 NH3 10 – 10 10 Biology -8 -7 -30 O3 10 – 10 3 x 10 Biology + photochemistry -7 -19 N2O 3 × 10 2 x 10 Biology (+minor abioc) Note: Number of molecules in Earth’s atmosphere ~1044 Bringing all gases to equilibrium (Gibbs energy minimizaon formal soluon): Gas Abundance Equilibrium Source Detectable in abundance Galileo NIR spectra of Earth? -6 -48 CH4 1.8 × 10 10 >90% biology; + geothermal -8 -7 -30 O3 10 – 10 2 x 10 Biology + photochemistry -7 -20 N2O 3 × 10 3 x 10 Biology (+minor abioc) Note: Number of molecules in Earth’s atmosphere ~1044 Assuming esmates of gas abundances…e.g., Radiave transfer ) sr model + retrieval/fit H O 2 CO H2O 2 Invert bands of well- /μm/ H2O CH4 H2O 2 N2O CO 2 mixed gas (i.e., CO2 CO 2 H2O H2O @4.3μm; 4.8μm) O3 H2O CO2 N2O Temperature- CH4 pressure profile Log flux (mW/cm wavelength (μm) CH4, N2O, H2O, O3 abundances, albeit Galileo Near-IR Mapping Spectrometer (Drossart+, 1993) non-unique vercal NIMS’ long-visible, O2 band @0.76 μm => column O2 I believe N2 was assumed by Sagan+ (1993). distribuon PART 2A: Does thermodynamic disequilibrium (in Gibbs energy/mole air) in Solar System atmospheres act as a metric for life? Part 2A Can quanfy chemical disequilibrium Atmosphere if it were in Observed atmosphere chemical equilibrium 15 Part 2A Can quanfy chemical disequilibrium Atmosphere if it were in Observed atmosphere chemical equilibrium moles gas i, ° Pγini G n (G RT ln( )) acvity coeff. γi (T ,P) = ∑ i i (T ,P ) + r n total moles air; do it for 1 i tot We quanfy disequilibrium as the change in Gibbs energy of the system during reacon to equilibrium: Available energy, ΔG = G (observed) − G (equilibrium) (T ,P) (T ,P) Applied to Solar System atmospheres…. 16 Part 1 Jupiter Available energy, ΔG= G(TT ,P) () obser− G( ,P) () equil ΔG =0.001J/mol Part 2A Mars O + 2CO ! 2CO 2 2 Available energy, ΔG= G(TT ,P) () obser− G( ,P) () equil ΔG =136 J/mol Venus Venus Available energy, ΔG= G(TT ,P) () obser− G( ,P) () equil ΔG =0.06 J/mol Part 2A Earth (atmosphere only) Available energy, 2O + CH ! CO + 2H O ΔG= G(TT ,P) () obser− G( ,P) () equil 2 4 2 2 ΔG =1.5 J/mol Typical surface of Mars Typical surface of planet Earth (2012: 13°S, mid-Atlantic, 3.8 km depth of water ) Photo on way to Ascension Island from St. Helena: David Catling Part 2A Earth (atmosphere-ocean fluid envelope) Atmospheric species Aqueous species Available energy, ΔG =2326 J/mol Gilbert Lewis (1923): “starting with air and water…nitric acid should form. It is to be hoped that nature will not discover a catalyst for this reaction, which would… turn the oceans into dilute nitric acid”. Part 2A Earth (atmosphere-ocean fluid envelope) Atmospheric species Aqueous species Available energy, ΔG =2326 J/mol 2N (g) + 5O (g) + 2H O(l) ! 4H + (aq) + 4NO− (aq) 2 2 2 3 Gilbert Lewis (1923): “starting with air and water…nitric acid should form. It is to be hoped that nature will not discover a catalyst for this reaction, which would…turn the oceans into dilute nitric acid”. Part 2A Earth has largest disequilibrium in the solar system Part 2A Earth has largest disequilibrium in the solar system Only on Earth is available energy ≈ thermal energy of air Part 2A Is this pracDcal for exoplanets? - For exoplanets, thermodynamic disequilibrium (in principle) could be computed from observaons without assumpons about gas fluxes. - Bulk abundance, oceans, and total pressure are observaonal challenges, but have been considered: - N2 from N2-N2 dimer absorpon, 4.3 μm (Schwieterman+ 2015). - Ocean presence from specular glint + H2O-rich spectra (e.g., Lack of glint on Mars: Phillips (1863) Proc. Roy Soc. Lond.; Sagan+ 1993; Robinson+ 2010; 2014) - Pressure from O2-O2 dimer, 1.06 & 1.27 μm (Misra+ 2014). - Sensivity tests to difficult-to-observe variables in the calculaon show relave insensivity Sensivity test Part 2A Sensivity test Part 2A Sensivity test Part 2A Sensivity test Part 2A Sensivity test Part 2A (extra slides) Part 2A What does disequilibrium mean? - Somemes thermodynamic disequilibrium means life. O N Biology: fixaon + nitrificaon 2 2 Biological nitrate Respiraon Oxygenic reducon photosynthesis (denitrificaon) CO + H O → O +CH O NO- 2 2 2 2 3 Part 2A What does disequilibrium mean? - Somemes thermodynamic disequilibrium means life. O N Biology: fixaon + nitrificaon 2 2 Biological nitrate Respiraon Oxygenic reducon photosynthesis (denitrificaon) CO + H O → O +CH O NO- 2 2 2 2 3 - But somemes thermodynamic disequilibrium means the absence of life (an-biosignature). Large available energy = an “uneaten free lunch” -> no life exists or “no one home” Mars Part 2A A primive metabolism: CO+ H222 O→ CO+ H Available energy, ΔG= G(TT ,P) () obser− G( ,P) () equil ΔG =136 J/mol Much CO => no life today on the surface of Mars. (Weiss et al. 2000; Zahnle et al. 2011). We calculate: ≤100 blue whales equiv. CO-eang biomass in subsurface. Part 2A What does disequilibrium mean? - Somemes thermodynamic disequilibrium means life. O N Biology: fixaon + nitrificaon 2 2 Biological nitrate Respiraon Oxygenic reducon photosynthesis (denitrificaon) CO + H O → O +CH O NO- 2 2 2 2 3 - But somemes thermodynamic disequilibrium means the absence of life: an-biosignature. Large available energy = an “uneaten free lunch” -> no life exists. - Conclusion: a single number metric like available energy has to be considered judiciously – in context. PART 2B (brief) KineDc metrics Part 2B Lifemes, sources, sinks ∂C Concentraon C; = −λt ⇒ C = C exp(−λt) => Lifeme = 1/λ ∂t 0 λ calculated from photochemical sinks. Examples below. abundance lifeDme Source Abioc (years) (Tmol/ fracon yr) CH4 1.8 ppmv 10 30 <10% N2O 0.3 ppmv 120 ~1 negligible To esmate source = sink, need to know: 1) CH4 + OH loss of CH4 1 2) Mainly N2O photolysis loss in stratosphere (plus some N2O + O( D)) To know OH sink on CH4 need to know… O(3P) O2 hv hv ground NO2 NO O3 state O2, N2 Muck: O(1D) oxidaon OH NH3, CO, H2S, SO2 excited ‘detergent’ CH4, organics NO2 H2O(g) rain 1 Keys: O3 and H2O(g) generate OH radicals; O3 photol.
Details
-
File Typepdf
-
Upload Time-
-
Content LanguagesEnglish
-
Upload UserAnonymous/Not logged-in
-
File Pages43 Page
-
File Size-