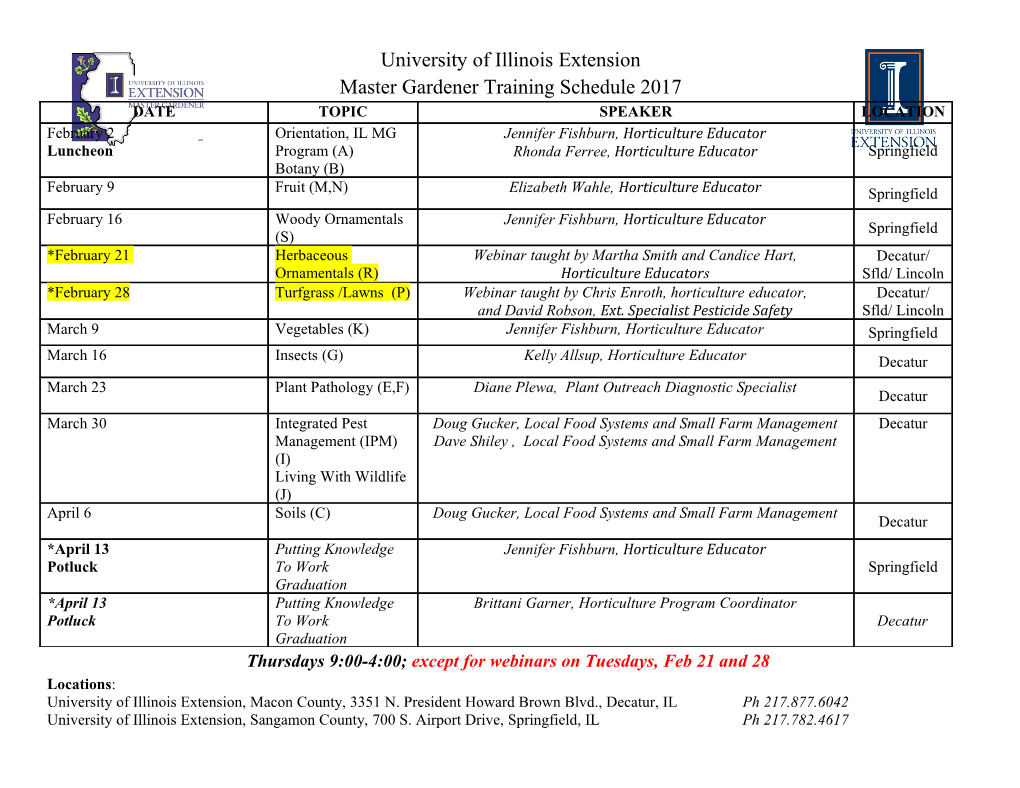
Earth Syst. Dynam. Discuss., https://doi.org/10.5194/esd-2018-84 Manuscript under review for journal Earth Syst. Dynam. Discussion started: 5 December 2018 c Author(s) 2018. CC BY 4.0 License. Climate feedbacks in the Earth system and prospects for their evaluation Christoph Heinze1,2, Veronika Eyring3,4, Pierre Friedlingstein5, Colin Jones6, Yves Balkanski7, Williams Collins8, Thierry Fichefet9, Shuang Gao1,#, Alex Hall10, Detelina Ivanova11,*, Wolfgang Knorr12, Reto 5 Knutti13, Alexander Löw14,†, Michael Ponater3, Martin G. Schultz15, Michael Schulz16, Pier Siebesma17,18, Joao Teixeira19, George Tselioudis20, and Martin Vancoppenolle21 1Geophysical Institute and Bjerknes Centre for Climate Research, University of Bergen, Postboks 7803, 5020 Bergen, Norway. 2Uni Research Climate, Bergen, Norway. 3Deutsches Zentrum für Luft- und Raumfahrt (DLR), Institut für Physik der Atmosphäre, Oberpfaffenhofen, Germany. 10 4University of Bremen, Institute of Environmental Physics (IUP), Bremen, Germany. 5College of Engineering, Mathematics and Physical Sciences, University of Exeter, Exeter, United Kingdom. 6National Centre for Atmospheric Science (NCAS), University of Leeds, Leeds, UK 7Laboratoire des Sciences du Climat et de l’Environnement, CEA/CNRS/UVSQ, Gif-sur-Yvette, France. 8Department of Meteorology, University of Reading, Reading, UK. 15 9Université catholique de Louvain, Earth and Life Institute, Georges Lemaître Centre for Earth and Climate Research, Louvain- la-Neuve, Belgium. 10Department of Atmospheric and Oceanic Sciences, University of California, Los Angeles, USA. 11Nansen Environmental and Remote Sensing Center (NERSC), Bergen, Norway. 12Department of Physical Geography and Ecosystem Science, Lund University, Lund, Sweden. 20 13Institute for Atmospheric and Climate Science, ETH Zürich, Zurich, Switzerland. 14Department for Geography, Ludwig Maximilian University, Munich, Germany. 15Forschungszentrum Jülich, Jülich, Germany. 16Norwegian Meteorological Institute, Oslo, Norway. 17Royal Netherlands Meteorological Institute, De Bilt, Netherlands. 25 18Department of Geoscience & Remote Sensing, Delft University of Technology, Delft, Netherlands. 19Jet Propulsion Laboratory, California Institute of Technology, Pasadena, California, USA. 20NASA Goddard Institute for Space Studies, New York City, USA. 21Sorbonne Universités (UPMC Paris 6), LOCEAN-IPSL, CNRS/ IRD/MNHN, Paris, France # Current address: Institute of Marine Research, Bergen, Norway 30 * Current address: Scripps Institution of Oceanography, La Jolla, USA. † deceased 2 July 2017 Correspondence to: Christoph Heinze ([email protected]) 35 Abstract. Earth system models (ESMs) are key tools for providing climate projections under different scenarios of human- induced forcing. ESMs include a large number of additional processes and feedbacks such as biogeochemical cycles that traditional physical climate models do not consider. Yet, some processes such as cloud dynamics and ecosystem functional response still have fairly high uncertainties. In this article, we present an overview of climate feedbacks for Earth system components currently included in state-of-the-art ESMs and discuss the challenges to evaluate and quantify them. Uncertainties 40 in feedback quantification arise from the interdependencies of biogeochemical matter fluxes and physical properties, the spatial and temporal heterogeneity of processes, and the lack of long-term continuous observational data to constrain them. We present an outlook for promising approaches that can help quantifying and constraining the large number of feedbacks in ESMs in the 1 Earth Syst. Dynam. Discuss., https://doi.org/10.5194/esd-2018-84 Manuscript under review for journal Earth Syst. Dynam. Discussion started: 5 December 2018 c Author(s) 2018. CC BY 4.0 License. future. The target group for this article includes generalists with a background in natural sciences and an interest in climate change as well as experts working in interdisciplinary climate research (researchers, lecturers, and students). This study updates and significantly expands upon the last comprehensive overview of climate feedbacks in ESMs, which was produced 15 years ago (NRC, 2003). 5 Outline / Table of contents page 1. Introduction: the Earth system model dilemma – complexity vs. uncertainty 3 2. From traditional climate feedbacks to Earth system feedbacks: what is forcing and what is 5 the system response? 2.1 Climate sensitivity and feedbacks in a purely physical climate model 5 2.2 Climate sensitivity, transient climate response, and feedbacks in an Earth system model 8 2.3 Choosing a reference forcing for Earth system feedbacks 11 3. Summary of fast physical climate feedbacks 13 3.1 Atmospheric thermodynamic feedbacks 13 3.2 Cloud feedbacks 17 3.3 Fast land surface feedbacks 20 3.4 Fast ocean feedbacks 21 3.5 Sea ice feedbacks 23 4. Introducing Earth system feedbacks 25 4.1 Slow vegetation–land surface–climate feedbacks 25 4.2 Slow ocean–atmosphere feedbacks 27 4.3 Land biogeochemistry feedbacks 28 4.4 Marine biogeochemical feedbacks 32 4.5 Aerosol–climate feedbacks 35 4.6 Tropospheric gas-phase chemistry feedbacks 38 4.7 Stratospheric composition feedbacks 42 5. Feedback evaluation outlook 44 5.1 ESM forcing and feedback evaluation within the model world 44 5.2 ESM feedback evaluation involving observations 48 6. Discussion 52 7. Conclusion 54 Acknowledgements 55 2 Earth Syst. Dynam. Discuss., https://doi.org/10.5194/esd-2018-84 Manuscript under review for journal Earth Syst. Dynam. Discussion started: 5 December 2018 c Author(s) 2018. CC BY 4.0 License. Appendix 1 Observational basis for feedback evaluation 56 Appendix 2 Glossary 66 Appendix 3 Abbreviations 69 References 70 1 Introduction: the Earth system model dilemma – complexity vs. uncertainty Anthropogenic emissions of greenhouse gases (GHGs) and aerosols (as well as respective precursor tracers) have altered the radiative balance of the Earth and induce changes in the climate on top of natural variations (IPCC, 2013). International 5 negotiations agreed on keeping the maximum global increase of global mean surface temperatures below +2°C relative to pre- industrial levels through reductions in GHG emissions (see discussions in Randalls (2010) and Knutti et al. (2015)), while the signatory countries pledged to make efforts to keep warming below +1.5°C (UNFCCC, 2015). In recent years, many atmosphere–ocean general circulation models (AOGCMs) have been extended to Earth system models (ESMs) that are used to project the extent, characteristics, and timing of climate change under given a future scenarios (ENES, 2012). ESMs also 10 contribute to the design of feasible mitigation pathways (e.g. through computation of allowable emissions in order to achieve a certain climate target; Ciais et al. (2013); Collins et al. (2013)). ESMs are climate models, which in addition to physical processes, also simulate a range of relevant biogeochemical cycles (land–biosphere, ocean biogeochemistry, atmospheric chemistry, and aerosols). Special attention in current ESMs is given to the carbon cycle (Bretherton, 1985; Flato, 2011; Jones et al., 2016) (Figure 1). Compared to conventional, purely physical coupled AOGCMs, ESMs include more process 15 representations, variables, and also climate-relevant feedbacks on both short (instantaneous to few years) and long (decades to centuries to millennia) timescales. ESMs are being continuously expanded to include additional processes. For example, the ESMs which form part of the Coupled Model Intercomparison Project Phase 6 (CMIP6, Eyring et al. (2016a)) will for the first time include interactive ice sheets (Nowicki et al., 2016) and several models will have interactive chemistry and aerosols (Collins et al., 2017). Multi-model ensembles of ESM simulations driven by GHG emissions show a larger spread in 20 projections of climate variables (such as surface temperature; see Meehl et al. (2007a)) than do physics-only simulations driven by GHG concentrations. This increase in uncertainty is a result of simulating a bigger part of the climate system interactively, including the carbon cycle and atmospheric trace species. Such complex model simulations reveal prevailing deficiencies in our prognostic capability of the full Earth system that need to be overcome. How can we assess the quality of the ESM simulations and how might we eventually reduce uncertainties? From observations, 25 we have identified many of the physical and biogeochemical processes operating within the Earth system, yet our understanding of these processes and their interactions on a global scale is still emerging. Observational data are often sparse, and observational time series rarely extend over climatological timescales. Many important processes or mechanisms in the 3 Earth Syst. Dynam. Discuss., https://doi.org/10.5194/esd-2018-84 Manuscript under review for journal Earth Syst. Dynam. Discussion started: 5 December 2018 c Author(s) 2018. CC BY 4.0 License. Earth system cannot be well constrained through measured parameters. Furthermore, many parameters of known relevance in the Earth system cannot be observed directly. The situation is particularly challenging for feedbacks acting on timescales longer than a decade due to sparse data coverage or a lack of high-quality measurements from the instrumental record. The lack of observational constraints underlines the need for employing models in order to make any useful statement about the 5 future evolution of the climate system at all. This presents
Details
-
File Typepdf
-
Upload Time-
-
Content LanguagesEnglish
-
Upload UserAnonymous/Not logged-in
-
File Pages94 Page
-
File Size-