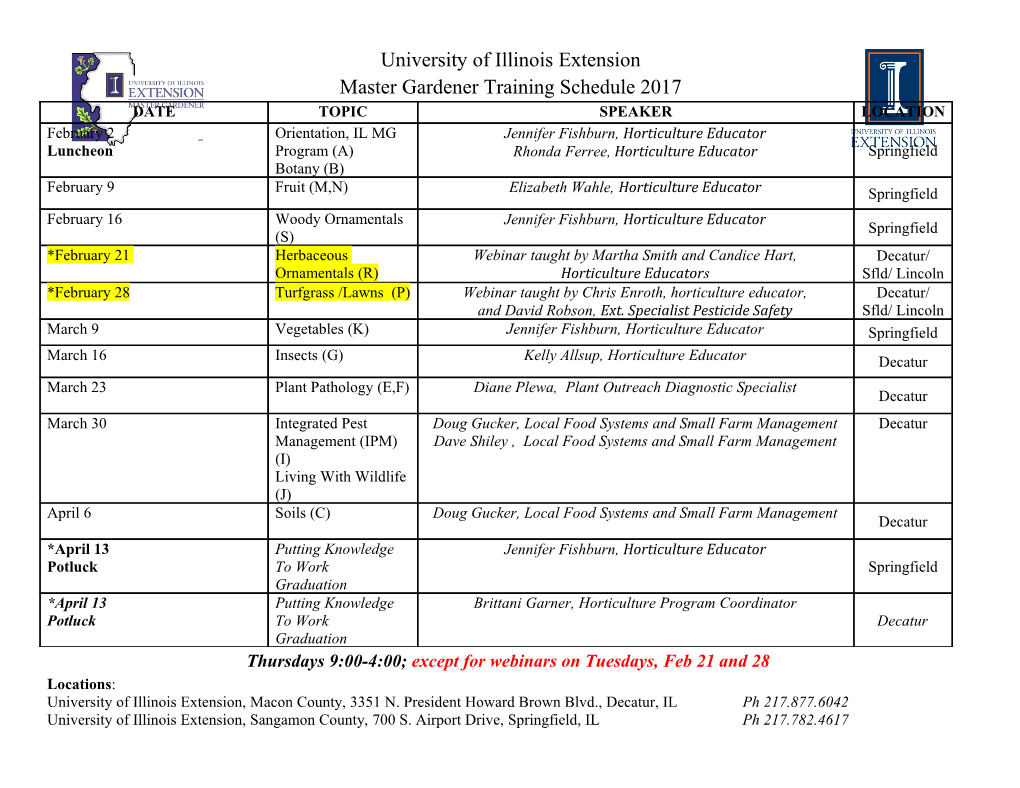
Lecture Notes in Functional Analysis Please report any mistakes, misprints or comments to [email protected] LECTURE 1 Banach spaces 1.1. Introducton to Banach Spaces Definition 1.1. Let X be a K{vector space. A functional p X 0; is called a seminorm, if (a) p λx λ p x ; λ K; x X, ∶ → [ +∞) (b) p x y p x p y ; x; y X. ( ) = S S ( ) ∀ ∈ ∈ Definition 1.2. Let p be a seminorm such that p x 0 x 0. Then, p is a norm ( + ) ≤ ( ) + ( ) ∀ ∈ (denoted by ). ( ) = ⇒ = Definition 1.3. A pair X; is called a normed linear space. Y ⋅ Y Lemma 1.4. Each normed space X; is a metric space X; d with a metric given by ( Y ⋅ Y) d x; y x y . ( Y ⋅ Y) ( ) Definition 1.5. A sequence x in a normed space X; is called a Cauchy se- ( ) = Y − Y n n N quence, if ∈ " 0 N{ " } N n; m N " (xn Yx⋅ mY) ": Definition 1.6. A sequence x converges to x (which is denoted by lim x x), ∀ > ∃ (n) n∈ N ∀ ≥ ( ) ⇒ Y − Y ≤ n n if ∈ →+∞ " 0 { N}" N n N " xn x ": = Definition 1.7. If every Cauchy sequence x converges in X, then X; is called ∀ > ∃ ( ) ∈ ∀ ≥n n (N ) ⇒ Y − Y < a complete space. { } ∈ ( Y ⋅ Y) Definition 1.8. A normed linear space X; which is complete is called a Banach space. ( 1 Y ⋅ Y) 2 LECTURE NOTES, FUNCTIONAL ANALYSIS Lemma 1.9. Let X; be a Banach space and U be a closed linear subspace of X. Then, U; is a Banach space as well. ( Y ⋅ Y) 1.2. Examples( Y ⋅ Y) of Banach spaces Example 1. Let B T be a space consisting of all bounded maps x T K. For each x B T we set ( ) x sup x t : ∶ Ð→ ∈ ( ) t T ∞ Then, B T ; is a Banach space.Y Y To= prove∈ S ( the)S assertion we need to show that (a) is a∞ norm, (b)( each( ) CauchyY ⋅ Y ) sequence converges to an element from B T . Y ⋅ Y∞ Concerning claim (a), let λ K and x B T . Then, ( ) (1.10) λx sup λ x t λ sup x t λ x : ∈ t T ∈ ( ) t T ∞ ∞ Let to T and x; y BY TY .= Then,∈ S ⋅ ( )S = S S ⋅ ∈ S ( )S = Y Y x to y to x to y to sup x t sup y t x y : ∈ ∈ ( ) t T t T ∞ ∞ The right handS ( ) side+ ( of)S the≤ S inequality( )S + S ( above)S ≤ is∈ independentS ( )S + ∈ S on( )St.= Therefore,Y Y + Y Y taking supre- mum of both sides of the inequality over t T yields (1.11) x y x y : ∈ Finally, let x be such that x 0, which is equivalent to sup x t 0. This implies Y + Y∞ ≤ Y Y∞ + Y Y∞ t T that x t 0 for each t. Thus, x 0. Y Y∞ = ∈ S ( )S = Now,S we( )S shall= prove the statement= (b). Let xn be a Cauchy sequence. Then, for every " 0 there exists N N " such that for all n; m N it holds that xn xm ". In particular, { } ∞ > = ( ) xn t xm t "; ≥ t T: Y − Y < Thus, for any t T the sequence xn t converges to some x t , due to the completeness S ( ) − n N( )S < ∀ ∈ of K (real and complex numbers are complete spaces). Define a candidate for a limit of the sequence x∈ , that is, x{ T ( )} ∈ as ( ) n n N K ∈ x t lim xn t : { } ∶ Ð→ n If follows from the statement above that( ) = there→+∞ exists( )No No "; t such that (1.12) x t x t "; n N : n =o ( ) Without loss of generality we can assume that N "; t N " for each t T . Then, for S ( ) − ( )S < o∀ ≥ n N it holds that ( ) ≥ ( ) ∈ x t x t x t x t x t x t ≥ n n No ";t No ";t xn xN ";t( ) " 2"; ( ) S ( ) − ( )S ≤ T ( ) − o ( )T + T ( ) − ( )T ( ) ≤ Y − Y∞ + < LECTURE 1. BANACH SPACES 3 where we used the fact that x is a Cauchy sequence and (1.12). Moreover, for each n n N t T and N N " we have ∈ x t { x} t x t x t x 2"; ∈ = ( ) N N N which implies x x 2" and so forth x B T . S N( )S ≤ S ( )S + S ( ) − ( )S ≤ Y Y∞ + Example 2. Let T be a metric space and C T be a space of bounded continuous func- Y Y∞ ≤ Y Y∞ + b ∈ ( ) tions on T . Then, Cb; is a Banach space. ( ) ∞ To prove the assertion,( Y it⋅ Y is) sufficient to show that Cb T is a closed subspace of B T (due to the Lemma 1.9), that is, to show that every sequence in Cb T which converges in B T converges to a point from C T . Let x be a( sequence) of bounded, continuous( ) b n n N functions convergent to x B T . We need to show that x is a continuous( ) function. For ∈ any( )" 0 there exists N N " ( N), such{ that} xN x " 3, since the sequence is convergent. Now, let to T∈. By( the) continuity of xN , there exists δ δ "; to 0 such that ∞ > d t;= t ( )δ ∈ x t Y x −t Y <" 3~: ∈ o N N o = ( ) > Therefore, for all t such that d t; t δ it holds that ( ) < o Ô⇒ S ( ) − ( )S < ~ x t x t x t x t x t x t x t x t o ( ) <N N N o N o o 2 x xN xN t xN to 2 " 3 " 3 "; S ( ) − ( )S ≤ S ( ) − ( )S + S ( ) − ( )S + S ( ) − ( )S which ends the proof. ≤ Y − Y∞ + S ( ) − ( )S ≤ ⋅ ~ + ~ = Example 3. A space of continuous functions vanishing at infinity n n Co R f Cb R lim f t 0 t with a norm is a Banach( ) space.= ∈ ( ) ∶ S S→+∞ S ( )S = Example 4. The following spaces Y ⋅ Y∞ co tn n tn ; lim tn 0 ; N K n ∈ c tn n tn ; lim→+∞ tn exists = { } N ∶ ∈ K n = ∈ with a norm are Banach= spaces.{ } ∶ ∈ →+∞ Remark 1. B is often denoted by l . Y ⋅ Y∞ N ∞ 1.3. lp spaces( ) Definition 1.13. Let 1 p . We define p ≤ < +∞ p p p l x l xn and x xn : +∞ p ¿+∞ ∞ n 1 Án 1 ÀÁ = ∈ ∶ Q= S S < +∞¡ Y Y = Q= S S 1 1 Lemma 1.14. (H¨olderinequality for sequences). Let 1 p; q be such that p q 1. Then, for x lp and y lq it holds that ≤ ≤ +∞ + = ∈ ∈ 4 LECTURE NOTES, FUNCTIONAL ANALYSIS (a) x y l1, (b) x y 1 x p y q. ⋅ ∈ p LemmaY 1.15.⋅ Y ≤(MinkowskiY Y ⋅ Y Y inequality for sequences). Let 1 p and x; y l . Then x y x y : p p p ≤ ≤ +∞ ∈ p Example 5. Spaces l ; p areY Banach+ Y ≤ spacesY Y + forY Y 1 p . Space l ; coincides Y ⋅ withY B N ; , therefore≤ we≤ assume+∞ that p . Similarly as in the∞ Example 1, to prove the assertion we need to show that ( Y ⋅ Y∞) ( ( ) Y ⋅ Y∞) < +∞ (a) p is a norm, (b) each Cauchy sequence converges to an element from lp. Claim (a)Y ⋅ isY straightforward, when one uses the Minkowski inequality for the proof of the triangle inequality. For the proof of completness, consider a Cauchy sequence xn . n N n p n n n p Each element x l is a sequence given by x x1 ; x2 ;::: . Note that l l , what ∈ holds due to the following estimate {∞ } ∈ = ( ) ⊂ p p p p x p xk sup xk sup xk x ; ¿+∞ k k Ák 1 ½ N N ÀÁ ∞ for x x ; x ;::: . Thus,Y Y = if weQ consider= S S ≥ the∈ sequenceS S = ∈xnS S =asY aY sequence of elements of 1 2 n N n l space, we conclude that there exists exactly one x l such that limn x x 0 ∈ (this∞ = follows( from) the completeness of l ; ). In{ particular,∞ } →+∞ ∞ n ∞ ∈ Y − Y = (1.16) lim x xk; for∞ each k : n k ( Y ⋅ Y ) N p n We shall show that x x →is+∞ an element of l space and that x converges to x k k N = ∈ n N in lp. For any " 0 there exists N N " , such that for all n; m N it holds that = { } ∈ { } ∈ xn xm ": > = ( ) p ≥ In particular, for every K N Y − Y < K ∈ p p xn xm xn xm ": ¿ k k p Ák 1 ÀÁ Q= T − T m≤ Y − Y < Using (1.16) and passing to the limit with xk we obtain K p p xn x ": ¿ k k Ák 1 ÀÁ Since the estimate is valid for all K andQ= theT right− handT < side of the inequality is independent on K, it holds also that p n p x xk ": ¿+∞ k Ák 1 ÀÁ Q= T − T < LECTURE 1. BANACH SPACES 5 n p Therefore x x p ", which proves that x is a limit of the sequence in l . Moreover, x x xN xN " xN : Y − Y < p p p p 1.4. Minkowski functionalY Y ≤ Y − Y + Y Y ≤ + Y Y < +∞ Definition 1.17. Set A is called an absorbing set if for each x X there exists t K, such that t x A. ∈ ∈ Definition 1.18. Set A is called a balanced set if x A x A. ⋅ ∈ Definition 1.19. Let A be a convex, absorbing and balanced set. A functional µ X ∈ ⇒ − ∈ A 0; defined by x ∶ Ð→ (1.20) µ x inf t 0; A [ +∞) A t is called Minkowski functional.( ) = ∈ ( +∞) ∶ ∈ Lemma 1.21.
Details
-
File Typepdf
-
Upload Time-
-
Content LanguagesEnglish
-
Upload UserAnonymous/Not logged-in
-
File Pages58 Page
-
File Size-