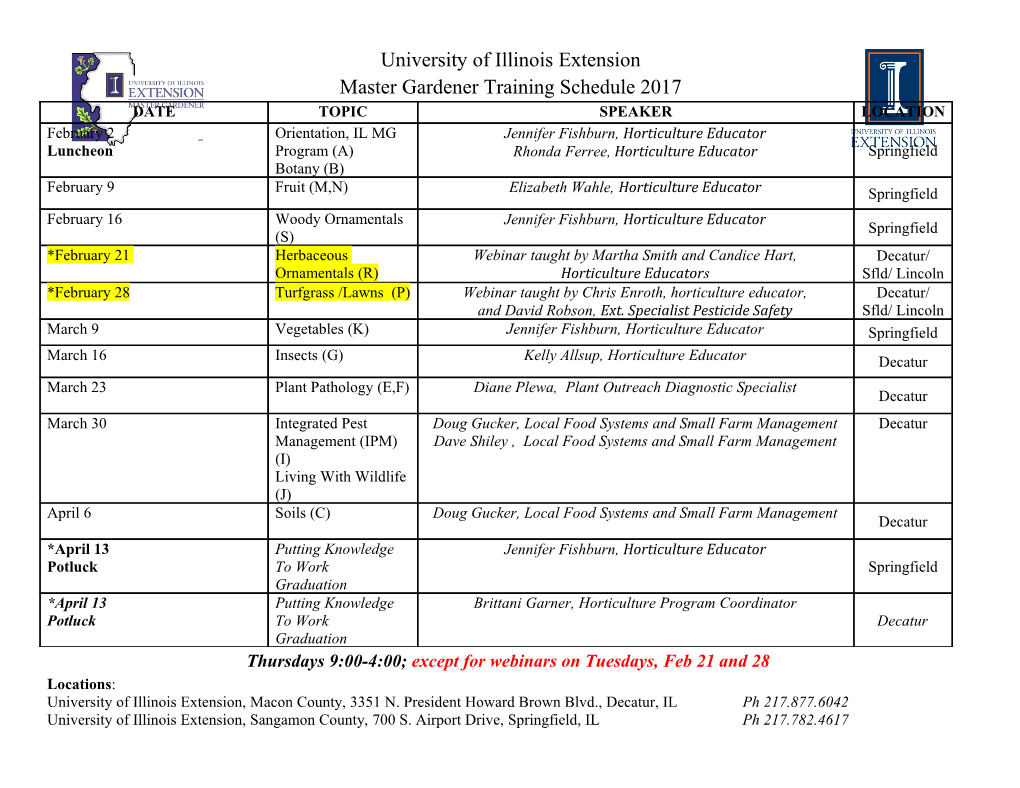
remote sensing Article Type III Radio Bursts Observations on 20th August 2017 and 9th September 2017 with LOFAR Bałdy Telescope Bartosz Dabrowski 1,* , Paweł Flisek 1 , Katarzyna Mikuła 1 , Adam Fro ´n 1 , Christian Vocks 2 , Jasmina Magdaleni´c 3,4 , Andrzej Krankowski 1 , PeiJin Zhang 5 , Pietro Zucca 6 and Gottfried Mann 2 1 Space Radio-Diagnostics Research Centre, University of Warmia and Mazury, 10-719 Olsztyn, Poland; pawel.fl[email protected] (P.F.); [email protected] (K.M.); [email protected] (A.F.); [email protected] (A.K.); 2 Leibniz-Institut für Astrophysik Potsdam (AIP), An der Sternwarte 16, 14482 Potsdam, Germany; [email protected] (C.V.); [email protected] (G.M.) 3 Solar-Terrestrial Centre of Excellence—SIDC, Royal Observatory of Belgium, 3 Avenue Circulaire, 1180 Uccle, Belgium; [email protected] 4 Center for mathematical Plasma Astrophysics, Department of Mathematics, KU Leuven, Celestijnenlaan 200B, B-3001 Leuven, Belgium 5 CAS Key Laboratory of Geospace Environment, School of Earth and Space Sciences, University of Science and Technology of China (USTC), Hefei 230026, China; [email protected] 6 ASTRON, The Netherlands Institute for Radio Astronomy, Oude Hoogeveensedijk 4, 7991PD Dwingeloo, The Netherlands; [email protected] * Correspondence: [email protected] Abstract: We present the observations of two type III solar radio events performed with LOFAR (LOw-Frequency ARray) station in Bałdy (PL612), Poland in single mode. The first event occurred on 20th August 2017 and the second one on 9th September 2017. Solar dynamic spectra were recorded in the 10 MHz up to 90 MHz frequency band. Together with the wide frequency bandwidth LOFAR telescope(with single station used) provides also high frequency and high sensitivity observations. Citation: Dabrowski, B.; Flisek, P.; Mikuła, K.; Fro´n,A.; Vocks, C.; Additionally to LOFAR observations, the data recorded by instruments on boards of the Interface Magdaleni´c,J.; Krankowski, A.; Region Imaging Spectrograph (IRIS) and Solar Dynamics Observatory (SDO) in the UV spectral range Zhang, P.; Zucca, P.; Mann, G. Type III complement observations in the radio field. Unfortunately, only the radio event from 9th September Radio Bursts Observations on 2017 was observed by both satellites. Our study shows that the LOFAR single station observations, 20th August 2017 and 9th September in combination with observations at other wavelengths can be very useful for better understanding 2017 with LOFAR Bałdy Telescope. of the environment in which the type III radio events occur. Remote Sens. 2021, 13, 148. https://doi.org/10.3390/rs13010148 Keywords: telescopes; LOFAR; IRIS; SDO; radio; UV; Sun Received: 29 October 2020 Accepted: 31 December 2020 Published: 5 January 2021 1. Introduction Publisher’s Note: MDPI stays neu- The type III bursts [1] belong to the most frequently observed radio events in the outer tral with regard to jurisdictional clai- layer of the Sun’s atmosphere—the corona. They are observed over a broad frequency ms in published maps and institutio- range, from 10 kHz up to 1 GHz. Type III bursts appear in groups of numerous bursts, nal affiliations. with total duration from few minutes up to a whole day or even longer, in case of a storm [2]. In dynamic spectra the type III bursts look like fast drifting, almost perpendicular structures. The small inclination indicates the direction of fast moving electron beams accelerated in the corona, which are responsible for the generation of type III bursts due to plasma Copyright: c 2021 by the authors. emission mechanism [3]. The relationship between the drift rate D f measured at the Licensee MDPI, Basel, Switzerland. frequency f and the radial source velocity Vr is given by: This article is an open access article distributed under the terms and con- f 1 dN D “ V (1) ditions of the Creative Commons At- f 2 N dr r tribution (CC BY) license (https:// creativecommons.org/licenses/by/ where N is the number density [4]. For type III bursts drift rates correspond to the 4.0/). electron beams velocity ranging typically between 0.1c and 0.6c [2](where c is the speed Remote Sens. 2021, 13, 148. https://doi.org/10.3390/rs13010148 https://www.mdpi.com/journal/remotesensing Remote Sens. 2021, 13, 148 2 of 17 of light). Drift rates decrease is directly proportional to the frequency and in the meter range are about several hundred MHz/s (see e.g., [5]). The duration of individual bursts varies inversely as a function of frequency. Time profiles of the type III bursts radio flux show rapid rise and slow decay. Additionally, decay seems to have an exponential distribution [6]. One of the characteristic features of a type III solar radio bursts is high drift rate, measured in MHz/s (see Table1). In the Figure1 we present drift rates as a function of frequency presented in publications listed in the Table1. The drift rate was limited up to 10–90 MHz frequency band, which corresponds to the frequency range of our research. The drift rates as a function of frequency obtained by [4,7] are similar, while the ones presented in [8] are somewhat different. Table 1. Drift rates for type III solar radio bursts. Drift (D f ) Frequency Range Reference [MHZ/S] [MHZ] ´0.01 f 1.84 0.075–550 [8] ´0.007354 f 1.76 0.04–85 [4] ´0.0672 f 1.23 10–80 [7] Figure 1. Compilation of drift rates versus frequency presented by different authors, listed in the Table1. The lines correspond to: Alvarez & Haddock, 1973 [ 8], Mann et al., 1999 [4] and Zhang et al., 2018 [7]. The negative value of the drift rates indicates that electron beams are moving upwards in the corona. For the proper velocity estimation of the electrons beams responsible for the type III solar radio bursts generation, besides the drift rate, knowledge about the electron density in the solar corona is also required. Several models of electron density in the solar corona, like for example [4,9–13], are currently in use. Four of them were presented in the Figure2. In this study, the radial velocity have been computed employing the two most commonly used electron density models proposed by [4,11]. Remote Sens. 2021, 13, 148 3 of 17 In the Mann et al. coronal density model [4] (hereafter called Mann et al. model) the radial velocity Vr is computed with the following formula: ´2 p´D f q f V “ ¨ v ¨ 1 ` 0.145 ln (2) r f 0 n f „ ˆ s ˙ where v0 “ 100, 620 km/s, f is the frequency, fs “ 644 MHz, n “ 1 (for the fundamental emission, n “ 2 would correspond to harmonic emission, but here we assume fundamental emission) and D f is the drift rate. In the Newkirk coronal density model [11] (hereafter called Newkirk model) the radial velocity Vr is expressed as: ´1 2D f d V “ ln pNq (3) r f dr ˆ ˙ where: d ´1 ´r2 ln pNq “ (4) dr 4.32 ¨ ln p10q ˆ ˙ where N is the number density, D f is the drift rate, f is the frequency and r is a distance from the center of the Sun in solar radius units[ 14]. For estimation of the type III radio burst parameters we assume they are emitted at the fundamental plasma frequency. The main objectives of our work was: (1) to determine the parameters of type III events, such as drift velocity, (2) to estimate the velocity of electrons responsible for the formation of such type of events and (3) to compare them with the results obtained by other authors. Furthermore, the additional goal was to add more detail to already established knowledge on type III radio bursts. For this task LOFAR telescope is extremely useful. This instrument is very sensitive with high frequency resolution that observes a broad bandwidth of very low radio frequencies (10–90 MHz). Such combination of parameters is extremely rare, hence the direct comparison of the results with other radio data sources is not always possible. For this purpose we used observations from a single LOFAR station in Bałdy (Poland). For better understanding of the conditions under which type III events are generated, we also used the data obtained by the IRIS and SDO satellites. Figure 2. Compilation of generally used models of electron density in the solar corona presented by different authors. The lines correspond to: Baumbach, 1937 [10], Allen, 1947 [9], Newkirk, 1961 [11], Saito et al., 1970 [13], Saito et al., 1977 [12] and Mann et al., 1999 [4]). Remote Sens. 2021, 13, 148 4 of 17 2. Instruments In this work we use the data from LOFAR, the LOw-Frequency ARray telescope (radio range), IRIS (ultraviolet range) and from SDO (ultraviolet range). 2.1. LOFAR Telescope LOFAR is a large radio interferometer working in a broad frequency range 10–240 MHz. LOFAR consists of over 50 stations, which are spread all over Europe (Figure3). The LOFAR “core” consists of 24 stations located near Exloo, The Netherlands. Six of these stations are placed on an artificial island with diameter of about 350 m called “Superterp”. 14 so called “remote” stations are located at a distance of up to 90 km from the core. In addition, LOFAR is extended with 14 “international” stations in Germany (6 stations), Poland (3 stations), and one station each in France, Sweden, United Kingdom, Ireland and Latvia. The largest baselines are of the order of 2000 kilometres, resulting in the 0.1 arc second resolution imaging in the 110–240 MHz range. The LOFAR stations are connected by a high speed network.
Details
-
File Typepdf
-
Upload Time-
-
Content LanguagesEnglish
-
Upload UserAnonymous/Not logged-in
-
File Pages17 Page
-
File Size-